Are you looking for a detailed comparison of deoxyhypusine synthase (DHS) structures? This vital enzyme plays a critical role in hypusination, a unique post-translational modification. At COMPARE.EDU.VN, we offer a comprehensive comparison of DHS structures to help researchers and students understand its function and implications in neurodegenerative diseases.
Deoxyhypusine synthase (DHS) is a critical enzyme involved in hypusination, a unique post-translational modification of eukaryotic translation initiation factor 5A (eIF5A). COMPARE.EDU.VN provides expert comparisons of DHS structures, offering key insights into enzyme mechanisms, disease-associated variants, and crucial cellular processes, thus helping you make well-informed decisions. Discover detailed analyses, structural comparisons, and functional implications related to DHS, including its role in protein synthesis and potential therapeutic interventions.
1. Introduction to Deoxyhypusine Synthase (DHS)
Deoxyhypusine synthase (DHS) is an essential enzyme responsible for catalyzing the first step in the hypusination pathway. This pathway is crucial for the function of eukaryotic translation initiation factor 5A (eIF5A), a protein involved in mRNA translation. Hypusination involves the post-translational modification of a specific lysine residue on eIF5A, which is critical for its role in protein synthesis and cell survival. Understanding the structure and function of DHS is vital for elucidating the mechanisms underlying hypusination and its implications in various cellular processes.
1.1. The Role of Hypusination
Hypusination is a unique post-translational modification that occurs on eIF5A. This modification involves the addition of a hypusine residue to a specific lysine residue on eIF5A. The hypusination process is essential for eIF5A’s function in protein synthesis, particularly in the translation of proteins containing polyproline stretches. Proper hypusination is critical for cell growth, proliferation, and survival.
1.2. Importance of DHS in Cellular Processes
DHS plays a pivotal role in the hypusination pathway by catalyzing the first committed step. This step involves the NAD-dependent transfer of the 4-aminobutyl moiety from spermidine to a specific lysine residue on eIF5A, forming deoxyhypusine. Given its role, DHS is essential for maintaining the proper level of hypusinated eIF5A, which affects a range of cellular processes including:
- Protein Synthesis: Facilitates the translation of specific mRNAs, especially those containing polyproline stretches.
- Cellular Growth and Proliferation: Supports cell division and growth by ensuring efficient protein production.
- Autophagy: Regulates autophagy, a critical process for cellular homeostasis.
- Neurodevelopment: Influences neuronal development and function, as evidenced by neurodevelopmental disorders associated with DHS mutations.
1.3. Clinical Relevance of DHS
Mutations in the DHPS gene, which encodes DHS, have been linked to severe neurodevelopmental disorders. Individuals with DHS deficiency often exhibit symptoms such as developmental delay, intellectual disability, and motor abnormalities. These clinical findings highlight the importance of DHS in normal neurological function. Understanding the structural and functional consequences of these mutations is crucial for developing potential therapeutic interventions.
1.4. Scope of the Comparison
This article compares different structural forms of DHS to provide a comprehensive understanding of its function and regulation. Specifically, it explores:
- Apoenzyme Structures: Structures of DHS without any bound ligands or substrates.
- Inhibitor-Bound Structures: Structures of DHS bound to inhibitors, such as GC7.
- Substrate-Bound Structures: Structures of DHS bound to its substrates, spermidine and eIF5A.
- Transition State Structures: Structures of DHS trapped in the reaction transition state.
- Disease-Associated Mutant Structures: Structures of DHS with mutations linked to neurodevelopmental disorders.
By comparing these different structures, this article aims to elucidate the conformational changes and molecular interactions that govern DHS activity, providing insights into the enzyme’s mechanism and potential therapeutic targets.
2. Overview of DHS Structures: Key Forms and Their Significance
Deoxyhypusine synthase (DHS) structures have been extensively studied through X-ray crystallography and cryo-electron microscopy (cryo-EM). Each structural form provides unique insights into DHS function, regulation, and the impact of disease-associated mutations. Here’s an overview of the key DHS structural forms and their significance:
2.1. Apoenzyme Structures
Apoenzyme structures represent DHS in its unbound state, without any substrates or inhibitors. These structures are crucial for understanding the basal conformation of the enzyme and identifying potential binding sites.
- Key Features: The apoenzyme structure reveals the overall architecture of DHS, including its tetrameric assembly. It highlights the composite active site formed by the interaction of two DHS protomers, which is essential for catalysis. The structure also shows the N-terminal ball-and-chain motif, which can block the entrance to the active site in the absence of substrate binding.
- Significance: By providing a baseline conformation, apoenzyme structures help researchers understand how substrate binding induces conformational changes that activate the enzyme. These structures are essential for structure-based drug design and understanding the mechanisms of enzyme regulation.
2.2. Inhibitor-Bound Structures
Inhibitor-bound structures of DHS provide insights into potential drug targets and the mechanisms of enzyme inhibition. One well-studied inhibitor is GC7, a spermidine analog that binds to the active site and blocks substrate binding.
- Key Features: The GC7-bound structure shows the inhibitor occupying the spermidine-binding pocket, preventing spermidine from binding and initiating the hypusination reaction. The structure reveals the specific interactions between GC7 and key residues in the active site, explaining the molecular basis of inhibition.
- Significance: Inhibitor-bound structures are valuable for developing new therapeutic agents that target DHS. They provide a detailed understanding of the active site and can guide the design of more potent and selective inhibitors.
2.3. Substrate-Bound Structures
Substrate-bound structures of DHS, including complexes with spermidine and eIF5A, are essential for understanding the catalytic mechanism. These structures show how the substrates bind to the active site and induce the conformational changes necessary for catalysis.
- Key Features: The spermidine-bound structure reveals the specific interactions between spermidine and key residues in the active site, which are critical for substrate recognition and binding. The eIF5A-DHS complex structure shows how eIF5A binds to DHS, positioning the target lysine residue for modification.
- Significance: Substrate-bound structures elucidate the molecular details of the catalytic mechanism, including the roles of specific residues in substrate binding, transition state stabilization, and product release. They provide a foundation for understanding how DHS activity is regulated and how mutations can disrupt its function.
2.4. Transition State Structures
Transition state structures capture DHS in the act of catalyzing the hypusination reaction. These structures provide direct evidence of the chemical steps involved in the reaction and the roles of specific residues in stabilizing the transition state.
- Key Features: Transition state structures show the formation of a covalent intermediate between spermidine and a lysine residue in the active site. They reveal the precise geometry of the reaction and the interactions that stabilize the transition state.
- Significance: Transition state structures provide critical insights into the enzyme’s catalytic mechanism. By understanding how DHS stabilizes the transition state, researchers can design more effective inhibitors that mimic the transition state and block enzyme activity.
2.5. Disease-Associated Mutant Structures
Structures of DHS mutants associated with neurodevelopmental disorders are crucial for understanding the molecular basis of these diseases. These structures reveal how specific mutations disrupt DHS structure and function, leading to impaired hypusination and neurological dysfunction.
- Key Features: Mutant structures may show altered active site conformations, impaired substrate binding, or disrupted tetramer assembly. These structural changes can explain the reduced catalytic activity and altered regulation observed in mutant enzymes.
- Significance: Disease-associated mutant structures provide a molecular understanding of the pathological mechanisms underlying DHS deficiency. This knowledge is essential for developing targeted therapies that correct the structural and functional defects caused by these mutations.
2.6. Comparative Significance
Each structural form of DHS provides unique information about its function and regulation. By comparing these structures, researchers can gain a comprehensive understanding of how DHS works and how mutations can disrupt its activity. This knowledge is crucial for developing effective treatments for DHS-related diseases. For instance, at COMPARE.EDU.VN, you can find detailed analyses of how specific mutations affect DHS structure and function, aiding in the development of targeted therapies.
3. Comparative Analysis of Apoenzyme Structures
Apoenzyme structures of deoxyhypusine synthase (DHS) provide fundamental insights into the enzyme’s architecture and regulatory mechanisms. These structures, devoid of substrates or inhibitors, offer a baseline understanding of the enzyme’s conformation in its resting state. Comparing different apoenzyme structures reveals key features and variations that influence DHS function.
3.1. Key Features of Apoenzyme Structures
- Overall Tetrameric Structure: DHS exists as a tetramer, comprising four identical subunits. Apoenzyme structures highlight this quaternary arrangement, showcasing how the subunits interact to form a stable and functional enzyme.
- Active Site Architecture: Each subunit contributes to an active site located at the interface between two protomers. The apoenzyme structure reveals the spatial arrangement of critical residues involved in substrate binding and catalysis.
- N-Terminal Ball-and-Chain Motif: This motif, present in each subunit, can block the entrance to the active site. The apoenzyme structure shows the position of this motif in its inhibitory conformation, providing insights into its regulatory role.
3.2. Structural Differences Among Apoenzyme Structures
While the overall architecture of DHS is conserved, subtle differences exist among various apoenzyme structures. These differences may arise from variations in crystallization conditions, protein constructs, or experimental techniques.
- Loop Conformations: Loop regions within DHS can exhibit varying degrees of order and flexibility. Comparing these loop conformations across different apoenzyme structures helps identify regions that undergo conformational changes upon substrate binding.
- Active Site Accessibility: The accessibility of the active site can vary among different apoenzyme structures. This variation may reflect differences in the position of the N-terminal ball-and-chain motif or other structural elements that regulate access to the active site.
- Subunit Interactions: Subtle differences in the interactions between DHS subunits can influence the overall stability and dynamics of the tetramer. Comparing these interactions across different apoenzyme structures provides insights into the factors that govern DHS assembly and stability.
3.3. Insights into Enzyme Regulation
Apoenzyme structures provide valuable insights into the mechanisms that regulate DHS activity. By revealing the enzyme’s resting state conformation, these structures help researchers understand how substrate binding and other regulatory factors can modulate DHS function.
- Role of the N-Terminal Ball-and-Chain Motif: The apoenzyme structure shows how this motif can block access to the active site, preventing substrate binding and inhibiting enzyme activity. This suggests that the motif plays a key role in regulating DHS activity by controlling access to the active site.
- Conformational Changes Upon Substrate Binding: By comparing apoenzyme structures with substrate-bound structures, researchers can identify the conformational changes that occur upon substrate binding. These changes may involve movements of loop regions, shifts in subunit interactions, or alterations in the position of the N-terminal ball-and-chain motif.
- Allosteric Regulation: While apoenzyme structures do not directly reveal allosteric regulatory mechanisms, they provide a foundation for understanding how allosteric effectors might bind to DHS and influence its activity. By identifying potential allosteric binding sites, researchers can design experiments to test the effects of various compounds on DHS function.
3.4. Comparative Examples
- Human DHS Apoenzyme Structure: This structure (PDB ID: 6XXJ) provides a high-resolution view of the DHS tetramer and its active site architecture.
- Mutant DHS Apoenzyme Structures: Structures of DHS mutants, such as those associated with neurodevelopmental disorders, can reveal how specific mutations disrupt the enzyme’s structure and function.
3.5. COMPARE.EDU.VN Resources
COMPARE.EDU.VN offers detailed comparisons of various DHS structures, including apoenzyme forms. These comparisons provide insights into the structural features and regulatory mechanisms of DHS, helping researchers understand the enzyme’s function and potential therapeutic targets.
- Structural Analysis Tools: COMPARE.EDU.VN provides tools for visualizing and comparing different DHS structures, allowing researchers to explore the subtle differences that influence enzyme activity.
- Expert Commentary: Our experts provide commentary on the significance of different structural features and their implications for DHS function and regulation.
- Disease-Associated Mutant Analysis: COMPARE.EDU.VN offers detailed analyses of DHS mutants associated with neurodevelopmental disorders, providing insights into the molecular basis of these diseases.
By leveraging the resources available at COMPARE.EDU.VN, researchers can gain a comprehensive understanding of DHS structure and function, accelerating the development of new therapies for DHS-related diseases.
4. Understanding Inhibitor-Bound Structures of DHS
Inhibitor-bound structures of deoxyhypusine synthase (DHS) are essential for designing and developing effective therapeutic agents. These structures provide a detailed view of how inhibitors interact with the enzyme, blocking its activity and preventing the hypusination of eIF5A. By comparing different inhibitor-bound structures, researchers can gain insights into the key interactions that govern inhibitor binding and selectivity.
4.1. Significance of Inhibitor-Bound Structures
- Drug Design: Inhibitor-bound structures provide a foundation for structure-based drug design, allowing researchers to design molecules that specifically target the active site of DHS and inhibit its activity.
- Mechanism of Inhibition: These structures reveal the molecular details of how inhibitors block DHS activity, including the specific interactions between the inhibitor and key residues in the active site.
- Selectivity and Specificity: By comparing the binding modes of different inhibitors, researchers can identify the structural features that confer selectivity for DHS over other enzymes.
4.2. Key Inhibitors of DHS
Several inhibitors of DHS have been identified and characterized, including:
- GC7: A spermidine analog that binds to the active site of DHS and prevents substrate binding.
- Berberine: A natural compound with inhibitory activity against DHS.
- Various Synthetic Inhibitors: Researchers have designed and synthesized numerous compounds with inhibitory activity against DHS, based on the structure of the active site.
4.3. Structural Features of Inhibitor Binding
Inhibitor-bound structures of DHS reveal several key features that govern inhibitor binding and selectivity:
- Active Site Interactions: Inhibitors typically bind to the active site of DHS, occupying the spermidine-binding pocket and preventing spermidine from binding. The structure shows the specific interactions between the inhibitor and key residues in the active site, such as hydrogen bonds, hydrophobic interactions, and electrostatic interactions.
- Conformational Changes: Inhibitor binding can induce conformational changes in the active site, which can either enhance or disrupt inhibitor binding. Understanding these conformational changes is essential for designing more effective inhibitors.
- Selectivity Determinants: By comparing the binding modes of different inhibitors, researchers can identify the structural features that confer selectivity for DHS over other enzymes. These features may include specific interactions with residues unique to the DHS active site or conformational changes that are only induced by binding to DHS.
4.4. Comparative Examples
- GC7-Bound DHS Structure: This structure (PDB ID: 2C1Q) shows GC7 occupying the spermidine-binding pocket, preventing spermidine from binding and initiating the hypusination reaction.
- Berberine-Bound DHS Structure: Structures of DHS bound to berberine reveal the specific interactions between the inhibitor and key residues in the active site, providing insights into its mechanism of inhibition.
4.5. COMPARE.EDU.VN Resources
COMPARE.EDU.VN provides resources for analyzing and comparing inhibitor-bound structures of DHS, including:
- Structural Visualization Tools: Tools for visualizing and comparing different inhibitor-bound structures, allowing researchers to explore the subtle differences that influence inhibitor binding and selectivity.
- Interaction Analysis: Analysis of the specific interactions between inhibitors and key residues in the active site, providing insights into the molecular basis of inhibition.
- Structure-Based Drug Design Resources: Resources for structure-based drug design, including tools for identifying potential drug targets and designing molecules that specifically target the active site of DHS.
By leveraging the resources available at COMPARE.EDU.VN, researchers can gain a comprehensive understanding of DHS inhibitor binding and selectivity, accelerating the development of new therapeutic agents for DHS-related diseases. If you’re looking for ways to compare protein folding dynamics and how they interact with the active site, COMPARE.EDU.VN is an excellent place to start.
5. Analyzing Substrate-Bound Structures of DHS
Substrate-bound structures of deoxyhypusine synthase (DHS) are critical for understanding the enzyme’s catalytic mechanism. These structures capture the enzyme in complex with its substrates, spermidine and eIF5A, providing insights into how these substrates bind to the active site and undergo chemical transformation. Comparing different substrate-bound structures helps elucidate the precise steps of the hypusination reaction.
5.1. Significance of Substrate-Bound Structures
- Catalytic Mechanism: Substrate-bound structures reveal the molecular details of the catalytic mechanism, including the roles of specific residues in substrate binding, transition state stabilization, and product release.
- Substrate Recognition: These structures show how DHS recognizes and binds its substrates, spermidine and eIF5A, including the specific interactions that govern substrate binding.
- Conformational Changes: Substrate binding can induce conformational changes in DHS, which are essential for catalysis. Substrate-bound structures capture these changes, providing insights into the dynamic nature of the enzyme.
5.2. Key Substrates of DHS
DHS interacts with two key substrates:
- Spermidine: Provides the 4-aminobutyl moiety that is transferred to eIF5A.
- eIF5A: The protein that undergoes hypusination.
5.3. Structural Features of Substrate Binding
Substrate-bound structures of DHS reveal several key features that govern substrate binding and catalysis:
- Spermidine-Binding Pocket: Spermidine binds in a specific pocket within the active site, where it interacts with key residues that stabilize its position. The structure shows the specific interactions between spermidine and these residues, such as hydrogen bonds and hydrophobic interactions.
- eIF5A Interaction: eIF5A interacts with DHS through a complex network of interactions involving both the N-terminal and C-terminal domains of eIF5A. The structure shows how eIF5A positions the target lysine residue for modification.
- Active Site Conformation: Substrate binding induces conformational changes in the active site, which are essential for catalysis. These changes may involve movements of loop regions, shifts in subunit interactions, or alterations in the position of the N-terminal ball-and-chain motif.
5.4. Comparative Examples
- Spermidine-Bound DHS Structure: These structures reveal the specific interactions between spermidine and key residues in the active site, providing insights into substrate recognition and binding.
- eIF5A-DHS Complex Structure: The cryo-EM structure of the eIF5A-DHS complex (PDB ID: 8A0E) shows how eIF5A binds to DHS, positioning the target lysine residue for modification. This structure provides a detailed view of the interactions between eIF5A and DHS, including the specific residues involved in complex formation.
5.5. COMPARE.EDU.VN Resources
COMPARE.EDU.VN offers resources for analyzing and comparing substrate-bound structures of DHS, including:
- Interactive Visualization Tools: Tools for visualizing and comparing different substrate-bound structures, allowing researchers to explore the subtle differences that influence substrate binding and catalysis.
- Detailed Interaction Analysis: Analysis of the specific interactions between substrates and key residues in the active site, providing insights into the molecular basis of substrate recognition and catalysis.
- Conformational Change Analysis: Analysis of the conformational changes that occur upon substrate binding, providing insights into the dynamic nature of the enzyme and its catalytic mechanism.
By leveraging the resources available at COMPARE.EDU.VN, researchers can gain a comprehensive understanding of DHS substrate binding and catalysis, accelerating the development of new therapies for DHS-related diseases.
6. Exploring Transition State Structures of DHS
Transition state structures of deoxyhypusine synthase (DHS) provide crucial insights into the enzyme’s catalytic mechanism by capturing DHS during the chemical reaction. These structures offer direct evidence of the chemical steps involved in the hypusination reaction and the roles of specific residues in stabilizing the transition state.
6.1. Importance of Transition State Structures
- Catalytic Mechanism: Transition state structures elucidate the precise chemical steps involved in the hypusination reaction, including the roles of specific residues in stabilizing the transition state.
- Enzyme Dynamics: These structures offer insights into the dynamic nature of the enzyme during catalysis, capturing the conformational changes that occur as the reaction proceeds.
- Drug Design: Transition state structures can be used to design more effective inhibitors that mimic the transition state, blocking enzyme activity with high potency and selectivity.
6.2. Capturing the Transition State
Capturing the transition state of an enzymatic reaction is challenging because it is a transient and unstable state. Researchers use several techniques to trap and stabilize the transition state, including:
- Mechanism-Based Inhibitors: These inhibitors mimic the transition state and bind tightly to the enzyme, preventing it from completing the reaction.
- Slow Substrates: Using substrates that react slowly allows researchers to capture the enzyme in the transition state for a longer period.
- Mutations: Introducing mutations that slow down the reaction can also help stabilize the transition state.
6.3. Structural Features of the Transition State
Transition state structures of DHS reveal several key features that characterize the chemical reaction:
- Covalent Intermediate: The formation of a covalent intermediate between spermidine and a lysine residue in the active site is a key feature of the transition state. The structure shows the precise geometry of this intermediate and the interactions that stabilize it.
- Charge Distribution: The transition state is characterized by a specific charge distribution, which is stabilized by interactions with residues in the active site. The structure shows how these residues interact with the transition state to lower the activation energy of the reaction.
- Conformational Changes: The transition state is often associated with significant conformational changes in the enzyme, which are essential for catalysis. The structure captures these changes, providing insights into the dynamic nature of the enzyme during catalysis.
6.4. Comparative Examples
- DHS with Trapped Transition State: The crystal structure of DHS with a trapped transition state (PDB ID: 8A0G) shows the formation of a covalent intermediate between spermidine and a lysine residue in the active site. This structure provides direct evidence of the chemical steps involved in the hypusination reaction and the roles of specific residues in stabilizing the transition state.
6.5. COMPARE.EDU.VN Resources
COMPARE.EDU.VN offers resources for analyzing and comparing transition state structures of DHS, including:
- Interactive Visualization Tools: Tools for visualizing and comparing different transition state structures, allowing researchers to explore the subtle differences that influence enzyme activity.
- Reaction Coordinate Analysis: Analysis of the reaction coordinate, providing insights into the energetic barriers and key intermediates involved in the hypusination reaction.
- Inhibitor Design Resources: Resources for designing inhibitors that mimic the transition state, providing a rational approach to drug discovery.
By leveraging the resources available at COMPARE.EDU.VN, researchers can gain a comprehensive understanding of DHS catalysis, accelerating the development of new therapies for DHS-related diseases.
7. Impact of Disease-Associated Mutant Structures on DHS
Structures of deoxyhypusine synthase (DHS) mutants associated with neurodevelopmental disorders are crucial for understanding the molecular basis of these diseases. These structures reveal how specific mutations disrupt DHS structure and function, leading to impaired hypusination and neurological dysfunction.
7.1. Significance of Mutant Structures
- Disease Mechanisms: Mutant structures provide a molecular understanding of the pathological mechanisms underlying DHS deficiency, a rare genetic disorder characterized by developmental delay, intellectual disability, and motor abnormalities.
- Structure-Function Relationships: By comparing mutant structures with the wild-type structure, researchers can identify the specific structural changes that disrupt DHS function.
- Therapeutic Targets: Mutant structures can be used to identify potential therapeutic targets for correcting the structural and functional defects caused by these mutations.
7.2. Key Disease-Associated Mutations
Several mutations in the DHPS gene have been linked to neurodevelopmental disorders, including:
- N173S: A common mutation found in individuals with DHS deficiency.
- delY305_I306: A deletion mutation that disrupts the structure and function of DHS.
7.3. Structural Features of Disease-Associated Mutants
Structures of DHS mutants reveal several key features that explain their impaired function:
- Active Site Distortion: Mutations can distort the active site, disrupting substrate binding and catalysis. The structure shows how these mutations alter the position of key residues in the active site, affecting their ability to interact with spermidine and eIF5A.
- Stability and Folding: Mutations can affect the stability and folding of DHS, leading to misfolding and aggregation. The structure shows how these mutations disrupt the overall architecture of the enzyme, making it more prone to degradation.
- Tetramer Assembly: Mutations can disrupt the assembly of DHS tetramers, which are essential for enzyme activity. The structure shows how these mutations interfere with the interactions between DHS subunits, preventing the formation of functional tetramers.
7.4. Comparative Examples
- DHSN173S Structure: The structure of DHSN173S (PDB ID: 7A6T) shows that this mutation does not have a significant impact on overall protein structure but does affect the ball-and-chain motif and likely also impacts the interaction with eIF5A.
- DHSdelY305_I306: This deletion mutation disrupts the structure and function of DHS, leading to impaired hypusination.
7.5. COMPARE.EDU.VN Resources
COMPARE.EDU.VN offers resources for analyzing and comparing structures of disease-associated DHS mutants, including:
- Structural Visualization Tools: Tools for visualizing and comparing different mutant structures, allowing researchers to explore the subtle differences that influence enzyme activity.
- Mutation Analysis: Analysis of the specific effects of mutations on DHS structure and function, providing insights into the molecular basis of disease.
- Therapeutic Target Identification: Resources for identifying potential therapeutic targets for correcting the structural and functional defects caused by DHS mutations.
By leveraging the resources available at COMPARE.EDU.VN, researchers can gain a comprehensive understanding of DHS deficiency, accelerating the development of new therapies for this debilitating disorder.
8. Comparative Tables: Summarizing Key Structural Features
To provide a clear comparison of the different DHS structures, the following tables summarize key features:
8.1. Comparison of Apoenzyme Structures
Feature | Description |
---|---|
Overall Structure | Tetramer with each subunit contributing to an active site |
Active Site Architecture | Composite active site formed by two protomers |
N-Terminal Motif | Ball-and-chain motif blocks active site |
Loop Conformations | Variable degrees of order and flexibility |
Subunit Interactions | Influences stability and dynamics of the tetramer |
Regulatory Role | N-terminal motif regulates active site access |
Example Structure (Human) | PDB ID: 6XXJ |
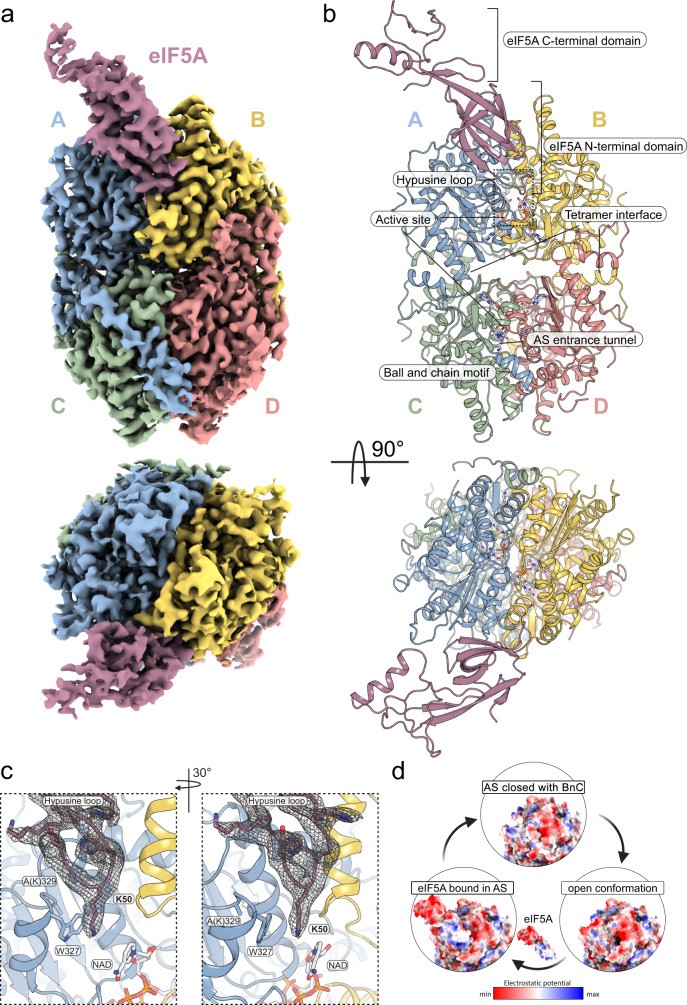
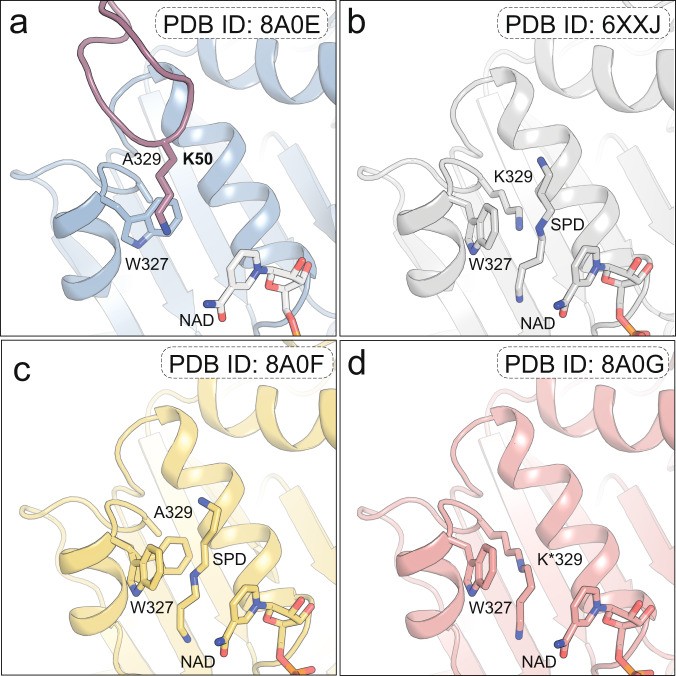
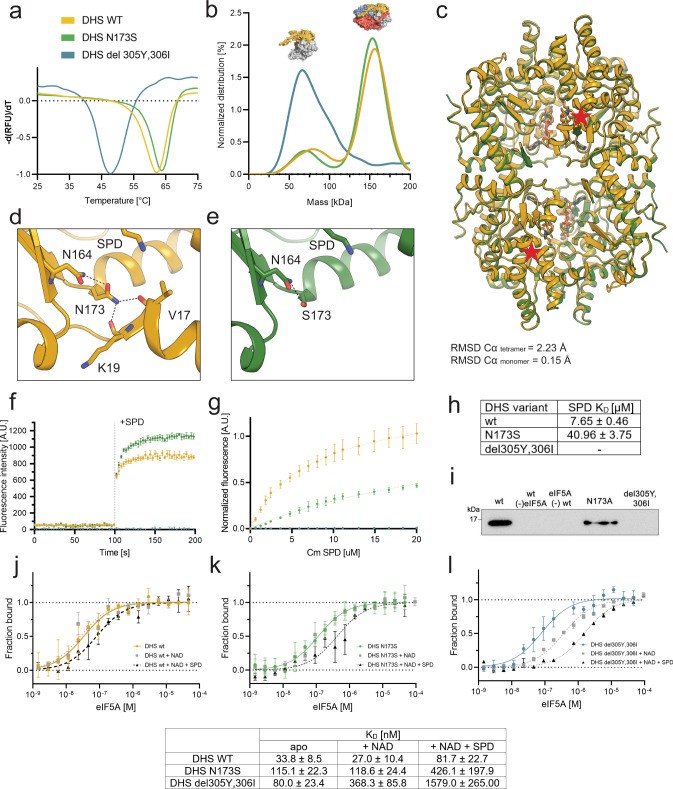
8.2. Comparison of Inhibitor-Bound Structures
Feature | Description |
---|---|
Inhibitor Binding | Occupies spermidine-binding pocket in active site |
Active Site | Interacts with key residues (hydrogen bonds, hydrophobic interactions) |
Conformational Changes | Can induce conformational changes in the active site |
Selectivity | Specific interactions with residues unique to DHS active site |
Example (GC7-Bound) | PDB ID: 2C1Q |
8.3. Comparison of Substrate-Bound Structures
Feature | Description |
---|---|
Spermidine Binding | Binds in specific pocket, interacts with key residues |
eIF5A Interaction | Interacts through N-terminal and C-terminal domains, positions target lysine |
Active Site | Induces conformational changes essential for catalysis (loop movements, subunit shifts) |
Example (eIF5A-DHS) | PDB ID: 8A0E (cryo-EM) |
8.4. Comparison of Transition State Structures
Feature | Description |
---|---|
Covalent | Formation of intermediate between spermidine and lysine |
Charge | Stabilized by interactions with active site residues |
Conformational | Changes essential for catalysis |
Example (Trapped) | PDB ID: 8A0G |
8.5. Comparison of Disease-Associated Mutant Structures
Feature | Description |
---|---|
Active Site | Distorted, affects substrate binding and catalysis |
Stability | Affects folding, leading to misfolding and aggregation |
Tetramer | Disrupts assembly of DHS tetramers |
Examples | N173S (PDB ID: 7A6T), delY305_I306 (disrupts structure and function) |
These tables provide a consolidated view of the structural features of DHS in various states, facilitating a comparative understanding of its function and regulation.
9. Frequently Asked Questions (FAQ) About DHS Structures
Here are some frequently asked questions about the structures of deoxyhypusine synthase (DHS):
Q1: What is the overall structure of DHS?
A1: DHS exists as a tetramer, comprising four identical subunits. Each subunit contributes to an active site located at the interface between two protomers.
Q2: What is the role of the N-terminal ball-and-chain motif in DHS?
A2: The N-terminal ball-and-chain motif can block the entrance to the active site, preventing substrate binding and inhibiting enzyme activity.
Q3: How do inhibitors bind to DHS?
A3: Inhibitors typically bind to the active site of DHS, occupying the spermidine-binding pocket and preventing spermidine from binding. They interact with key residues in the active site through hydrogen bonds, hydrophobic interactions, and electrostatic interactions.
Q4: What is the significance of the eIF5A-DHS complex structure?
A4: The eIF5A-DHS complex structure shows how eIF5A binds to DHS, positioning the target lysine residue for modification. It provides a detailed view of the interactions between eIF5A and DHS, including the specific residues involved in complex formation.
Q5: What do transition state structures of DHS reveal?
A5: Transition state structures of DHS reveal the precise chemical steps involved in the hypusination reaction, including the roles of specific residues in stabilizing the transition state.
Q6: How do disease-associated mutations affect DHS structure and function?
A6: Disease-associated mutations can distort the active site, affect the stability and folding of DHS, and disrupt the assembly of DHS tetramers, leading to impaired hypusination and neurological dysfunction.
Q7: What are some common mutations associated with DHS deficiency?
A7: Common mutations include N173S and delY305_I306, which have been linked to neurodevelopmental disorders.
Q8: How can structural information be used to develop new therapies for DHS-related diseases?
A8: Structural information can be used to design inhibitors that specifically target the active site of DHS, correct the structural and functional defects caused by mutations, and identify potential therapeutic targets for correcting the structural and functional defects caused by these mutations.
Q9: Where can I find detailed comparisons of DHS structures?
A9: COMPARE.EDU.VN offers detailed comparisons of various DHS structures, providing insights into the structural features and regulatory mechanisms of DHS.
Q10: How does DHS impact protein synthesis and cell survival?
A10: DHS plays a vital role in hypusination, which is essential for eIF5A’s function in protein synthesis, particularly in the translation of proteins containing polyproline stretches. Proper hypusination is critical for cell growth, proliferation, and survival.
10. Conclusion: Leveraging DHS Structural Knowledge for Therapeutic Advances
Understanding the different structures of deoxyhypusine synthase (DHS) is crucial for elucidating its function, regulation, and implications in neurodegenerative diseases. COMPARE.EDU.VN provides a comprehensive platform for comparing these structures, offering researchers and students invaluable insights into enzyme mechanisms, disease-associated variants, and potential therapeutic interventions.
By exploring apoenzyme structures, inhibitor-bound structures, substrate-bound structures, transition state structures, and disease-associated mutant structures, we gain a holistic view of DHS and its role in cellular processes. The comparative tables and expert analyses offered by COMPARE.EDU.VN further enhance this understanding, facilitating the development of targeted therapies for DHS-related disorders.
Take advantage of the resources at COMPARE.EDU.VN to delve deeper into DHS structural biology and contribute to advancements in treating neurodevelopmental diseases. For detailed structural analyses, access to interactive visualization tools, and expert commentary, visit COMPARE.EDU.VN today.
Ready to explore the world of DHS structures? Visit COMPARE.EDU.VN to access comprehensive comparisons, expert analysis, and interactive tools that will help you understand DHS and its role in neurodevelopmental disorders. Contact us at 333 Comparison Plaza, Choice City, CA 90210, United States, or reach out via Whatsapp at +1 (626) 555-9090. Let compare.edu.vn be your guide to making informed decisions and driving therapeutic advances.