Comparing earthquake magnitudes is crucial for understanding the potential impact of seismic events. COMPARE.EDU.VN offers a detailed guide to help you effectively compare earthquake magnitudes, understand the different scales used, and interpret the data for informed decision-making. This guide dives deep into magnitude types, energy release, intensity, and real-world examples, enabling you to grasp the complexities of seismic measurements and their implications.
1. Understanding Earthquake Magnitude
Earthquake magnitude is a numerical representation of the size or strength of an earthquake. This single value is determined from data recorded by seismometers, which detect and measure ground vibrations caused by seismic waves. Analyzing these seismograms provides essential information about the earthquake’s time, location, and magnitude. While magnitude represents the overall size, the shaking intensity varies from place to place, influenced by factors like distance, surface material, and geological conditions.
1.1 The Significance of Magnitude
Magnitude serves as a standardized measure allowing scientists and the public to compare the relative sizes of different earthquakes. This is vital for assessing potential damage and understanding the seismic activity of a region. While a higher magnitude indicates a larger earthquake, the actual ground shaking experienced at a specific location also depends on factors like distance from the epicenter and local geological conditions.
1.2 Types of Earthquake Magnitudes
Several magnitude scales are used to quantify earthquake size, each with its own methodology and applicability. Understanding these different scales is essential for accurately comparing earthquake magnitudes and interpreting the reported values.
2. Exploring Different Magnitude Scales
While initially believed to be equivalent, different magnitude scales can yield varying results, especially for very large earthquakes. Modern seismology relies on measurements that describe the physical effects of an earthquake rather than solely relying on waveform amplitudes.
2.1 The Richter Scale (ML)
The Richter Scale, developed by Charles F. Richter in 1935, was the first widely used method for measuring earthquake magnitude. It is based on the logarithm of the amplitude of seismic waves recorded on seismographs. Adjustments are made to account for the distance between seismographs and the earthquake epicenter.
2.1.1 Limitations of the Richter Scale
The Richter Scale is most accurate for shallow, local earthquakes. It tends to underestimate the magnitude of large earthquakes and is not suitable for distant events. As a result, it is now primarily used for small, local earthquakes where other magnitude scales may not be applicable.
2.2 The Moment Magnitude Scale (Mw)
The Moment Magnitude Scale (Mw) is currently the most widely used and reliable measure of earthquake magnitude, particularly for large earthquakes. It is based on the seismic moment, which is calculated from the physical properties of the earthquake, including the rigidity of the rock, the area of the fault that slipped, and the amount of slip.
2.2.1 Calculating Moment Magnitude
The seismic moment (Mo) is calculated using the formula:
Mo = rigidity x area x slip
Where:
- Rigidity: The strength of the rock along the fault.
- Area: The area of the fault that slipped during the earthquake.
- Slip: The distance the fault moved.
The moment magnitude (Mw) is then calculated using the formula:
Mw = (2/3) log10(Mo) – 9.1
2.2.2 Advantages of the Moment Magnitude Scale
The Moment Magnitude Scale provides a more accurate representation of earthquake size, especially for large earthquakes, as it is directly related to the physical properties of the earthquake rupture. It also overcomes the limitations of the Richter Scale, making it suitable for earthquakes of all sizes and distances.
2.3 Other Magnitude Scales
Besides the Richter Scale and the Moment Magnitude Scale, other magnitude scales are used in seismology, each tailored to specific types of seismic waves or earthquake characteristics. These include:
- Body Wave Magnitude (Mb): Measures the amplitude of body waves (waves that travel through the Earth’s interior).
- Surface Wave Magnitude (Ms): Measures the amplitude of surface waves (waves that travel along the Earth’s surface).
- Energy Magnitude (Me): Estimates the energy released by an earthquake.
2.4 Magnitude Types Table
Magnitude Type | Magnitude Range | Distance Range | Equation | Description |
---|---|---|---|---|
Richter Scale (ML) | Typically < 6.0 | Local | Logarithmic amplitude of seismic waves | Best for small, local earthquakes; underestimates large earthquakes. |
Moment Magnitude (Mw) | No limit | All distances | Mw = (2/3) log10(Mo) – 9.1 | Based on the seismic moment; provides the most accurate measure for all earthquake sizes. |
Body Wave Magnitude (Mb) | Typically < 6.5 | Distant | Based on amplitude of body waves | Useful for distant earthquakes, but saturates for large events. |
Surface Wave Magnitude (Ms) | Typically < 8.0 | Distant | Based on amplitude of surface waves | Used for shallow earthquakes, but less accurate for deep events and saturates for large events. |
Energy Magnitude (Me) | No limit | All distances | Log E = 5.24 + 1.44Mw | Estimates the energy released by the earthquake; related to Moment Magnitude but measures a different property, so values may differ. |
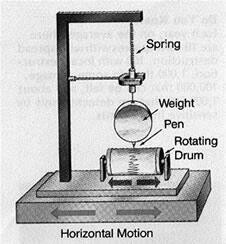
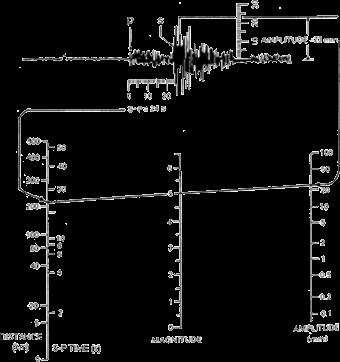
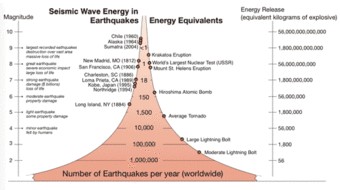
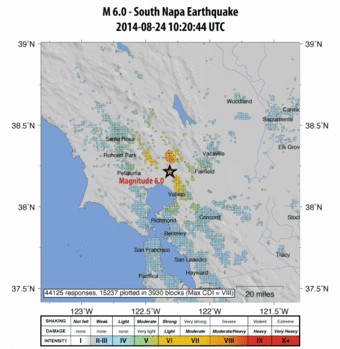
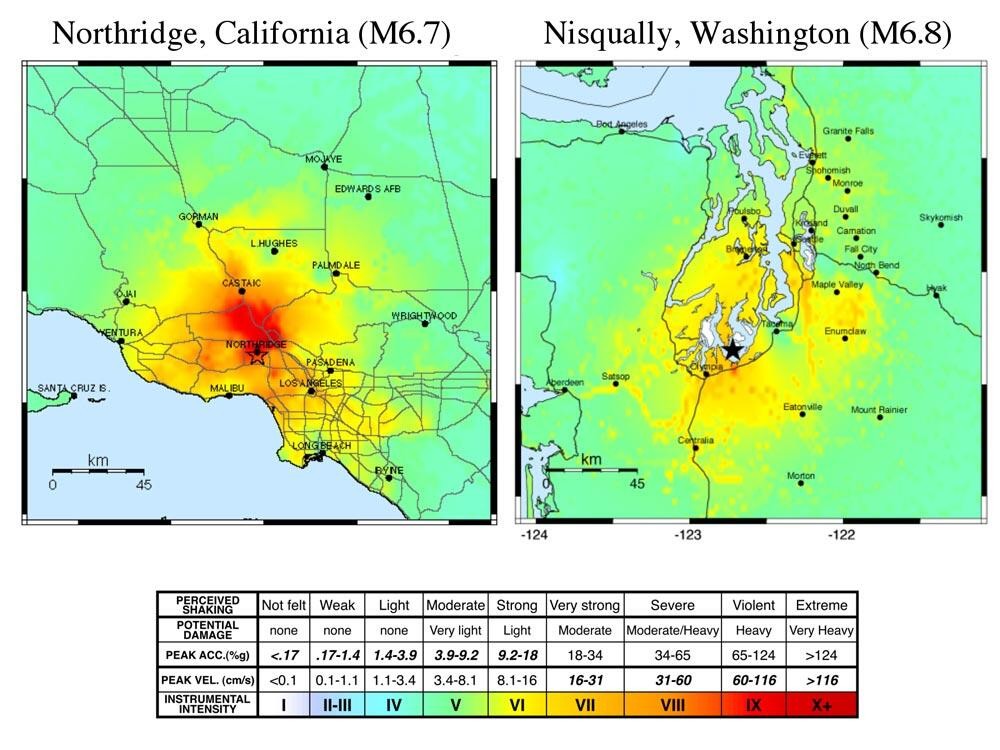
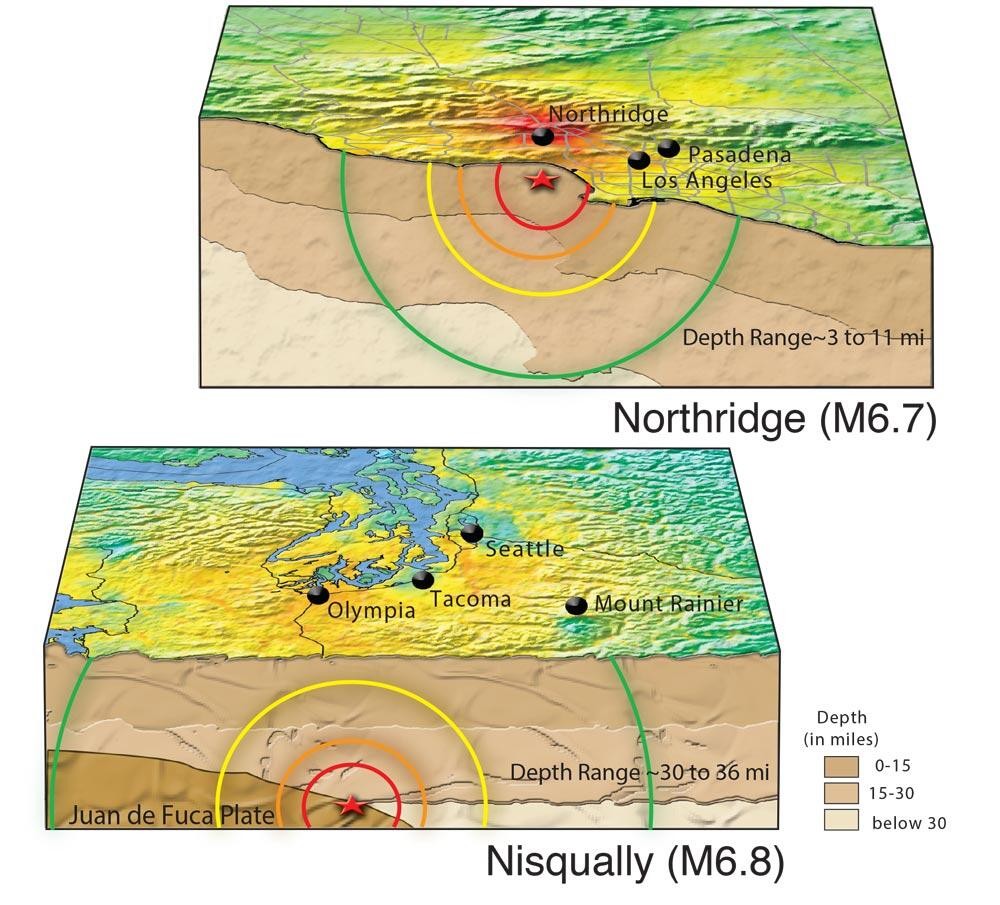
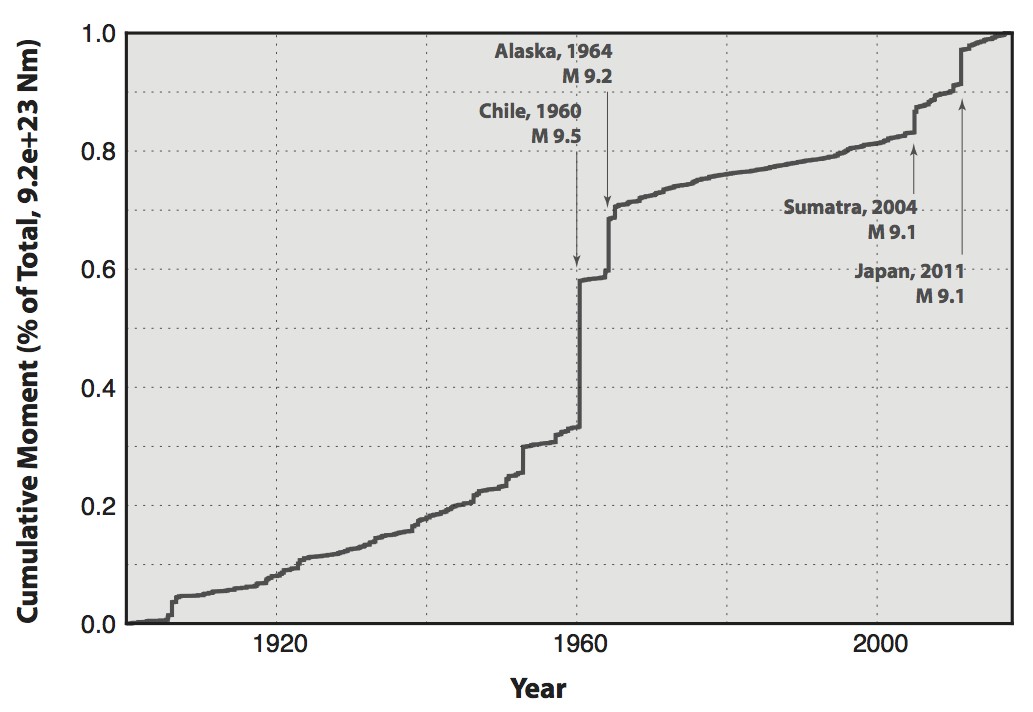
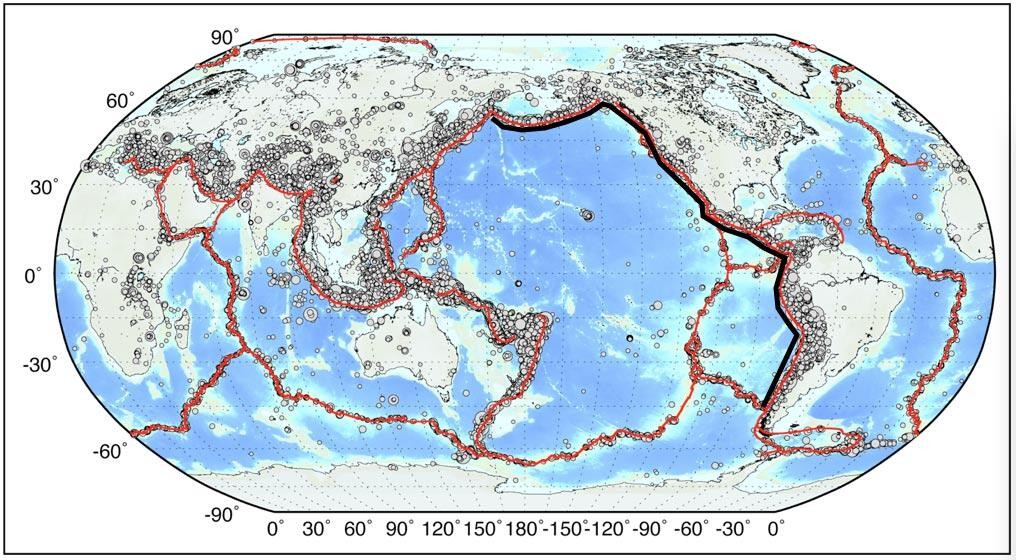
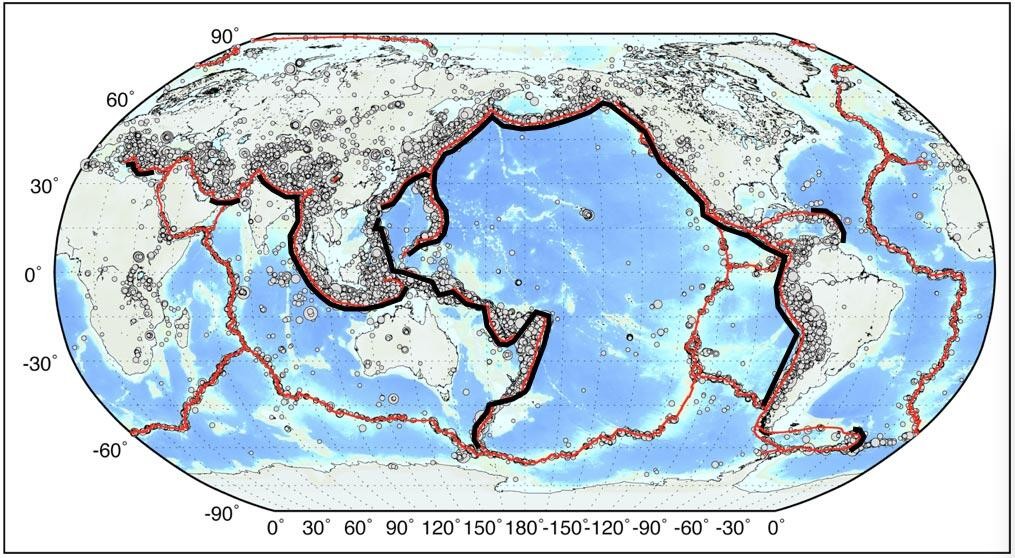
Understanding the different magnitude scales is essential for accurately comparing earthquake sizes and interpreting seismic data. Each scale has its strengths and limitations, making it crucial to consider the context when evaluating earthquake magnitudes.
3. Understanding Energy Release
While magnitude provides a measure of earthquake size, the energy released by an earthquake is a critical factor in determining its potential for damage. The relationship between magnitude and energy release is logarithmic, meaning that each whole number increase in magnitude represents a significant increase in energy.
3.1 The Logarithmic Relationship
Each whole number increase in magnitude represents approximately a tenfold increase in measured amplitude on a seismogram. However, it represents roughly 32 times more energy release. This exponential relationship highlights the vast difference in energy between earthquakes of even slightly different magnitudes.
3.2 Calculating Energy Release
The energy released by an earthquake can be estimated using the following equation:
log E = 5.24 + 1.44Mw
Where:
- E is the energy released in joules.
- Mw is the moment magnitude.
3.3 Comparing Energy Release Examples
To illustrate the difference in energy release, consider the following examples:
- A magnitude 6.0 earthquake releases approximately 1.79 x 10^13 joules of energy.
- A magnitude 7.0 earthquake releases approximately 5.62 x 10^14 joules of energy (about 32 times more than a magnitude 6.0 earthquake).
- A magnitude 8.0 earthquake releases approximately 1.77 x 10^16 joules of energy (about 32 times more than a magnitude 7.0 earthquake).
These examples demonstrate the immense difference in energy released by earthquakes of different magnitudes and highlight the importance of considering energy release when assessing the potential impact of seismic events.
4. Understanding Earthquake Intensity
While magnitude is a single value representing the overall size of an earthquake, intensity describes the degree of shaking at a specific location. Intensity varies depending on factors such as distance from the epicenter, local geology, and the earthquake’s rupture characteristics.
4.1 The Modified Mercalli Intensity Scale
The Modified Mercalli Intensity Scale (MMI) is commonly used in the United States to assess earthquake intensity. It is a subjective scale based on observations of felt shaking, damage, and other effects of the earthquake. Intensity values are expressed in Roman numerals, ranging from I (not felt) to XII (catastrophic damage).
4.2 Factors Affecting Intensity
Several factors influence the intensity of shaking at a particular location:
- Distance from the Epicenter: Intensity generally decreases with increasing distance from the earthquake’s epicenter.
- Local Geology: Soft soils and sediments tend to amplify shaking, while bedrock reduces it.
- Earthquake Depth: Shallow earthquakes typically produce higher intensities than deeper earthquakes of the same magnitude.
- Rupture Characteristics: The direction and extent of fault rupture can affect the distribution of shaking.
4.3 Comparing Magnitude and Intensity
It is important to distinguish between magnitude and intensity. Magnitude is a measure of the earthquake’s overall size, while intensity is a measure of the shaking experienced at a specific location. An earthquake has only one magnitude but many intensity values distributed across the affected area.
5. Examples Illustrating Magnitude and Intensity
To further clarify the concepts of magnitude and intensity, consider the following examples:
5.1 The Impact of Local Geology on Shaking
The Loma Prieta earthquake (M6.9) in 1989 demonstrated the influence of local geology on shaking intensity. Seismometers at different locations recorded varying levels of shaking, even at similar distances from the epicenter. Stations located on bedrock experienced the least shaking, while those on soft mud experienced the most.
5.2 The Impact of Earthquake Depth on Shaking
The Northridge, CA earthquake (M6.7) in 1994 and the Nisqually, WA earthquake (M6.8) in 2001 illustrate the effect of earthquake depth on shaking intensity. The Northridge earthquake, which occurred at a shallower depth, produced more intense shaking over a wider area than the Nisqually earthquake, which occurred at a greater depth.
5.3 Cumulative Moment Release
The small- and moderate-size earthquakes that occur frequently around the world release far less energy than a single great earthquake.
6. Hypothetical Earthquake Scenarios
To put earthquake magnitudes into perspective, let’s consider hypothetical scenarios and the fault characteristics required to generate such events.
6.1 The Energy Required for a Magnitude N Earthquake
Summing the energy released by all earthquakes over the past ~110 years yields an equivalent magnitude of approximately Mw 9.95.
6.2 Fault Rupture and Magnitude
If the San Andreas Fault were to rupture end-to-end (~1400km) with an average slip of ~10m, it would produce an earthquake of Mw 8.47. Similarly, if the South American subduction zone were to rupture end-to-end (~6400km) with an average slip of ~40m, it would result in an earthquake of Mw 9.86.
6.3 Hypothetical Magnitude 10 Earthquake
To generate an Mw 10 earthquake, you would need a fault length of approximately 14,000km with an average seismogenic thickness of 40km and an average slip of 30m.
6.4 Hypothetical Magnitude 10.5 Earthquake
Creating an Mw 10.5 earthquake would require ~80,000km of fault length with an average seismogenic width of 100km. Considering that all subduction zones in the world, along with adjoining structures, amount to ~40,000km (and the Earth’s circumference is also ~40,000km), an Mw 10.5 earthquake is highly unlikely.
7. FAQs on Comparing Earthquake Magnitudes
Here are some frequently asked questions about comparing earthquake magnitudes:
7.1 What is the difference between magnitude and intensity?
Magnitude measures the size of an earthquake, while intensity measures the shaking at a specific location.
7.2 Which magnitude scale is the most accurate?
The Moment Magnitude Scale (Mw) is generally considered the most accurate, especially for large earthquakes.
7.3 How much more energy is released with each magnitude increase?
Each whole number increase in magnitude represents approximately 32 times more energy release.
7.4 Why do earthquakes of the same magnitude cause different levels of damage?
Factors like earthquake depth, local geology, and building construction influence the amount of damage.
7.5 Can earthquakes have negative magnitudes?
While theoretically possible, earthquakes with negative magnitudes are extremely rare and typically very small.
7.6 How are earthquake magnitudes determined?
Earthquake magnitudes are determined by analyzing data from seismometers, which measure ground motion.
7.7 What is the significance of a high magnitude earthquake?
High magnitude earthquakes can cause significant damage, loss of life, and disruption to infrastructure.
7.8 Where can I find information about recent earthquakes?
Reliable sources include the USGS (United States Geological Survey) and other national and international seismological agencies.
7.9 How can I protect myself during an earthquake?
Follow the “Drop, Cover, and Hold On” protocol: drop to the ground, cover your head and neck, and hold on to a sturdy object.
7.10 How does COMPARE.EDU.VN help in understanding earthquake data?
COMPARE.EDU.VN provides comprehensive comparisons and explanations of earthquake magnitudes, intensity, and related concepts to help users better understand seismic events.
8. Conclusion: Making Sense of Earthquake Data
Understanding How To Compare Earthquake Magnitudes is essential for assessing potential risks and understanding the science behind these natural phenomena. By understanding the different magnitude scales, the relationship between magnitude and energy release, and the factors influencing intensity, you can make informed decisions based on earthquake data.
Navigating the complexities of earthquake data can be challenging. If you’re looking for clear, objective comparisons to aid in your understanding and decision-making, visit COMPARE.EDU.VN. We offer detailed analyses and insights to help you make sense of seismic events and their potential impacts.
For further information, contact us at:
Address: 333 Comparison Plaza, Choice City, CA 90210, United States
WhatsApp: +1 (626) 555-9090
Website: compare.edu.vn