How To Compare Boiling Points? This is a common question in chemistry, and COMPARE.EDU.VN offers a clear understanding of this concept. Understanding the factors that influence boiling points, such as intermolecular forces and molecular weight, helps you make informed decisions in various scientific contexts. Delve into the world of comparing boiling points, boiling temperature, and thermal stability with COMPARE.EDU.VN, your trusted source for insightful comparisons.
1. Understanding Boiling Point Trends: An Introduction
Boiling point determination hinges on recognizing fundamental trends. It’s the energy required to transform a substance from its liquid phase into a gaseous phase. The strength of intermolecular forces dictates the magnitude of the boiling point. Stronger intermolecular forces equate to higher boiling points due to the increased energy needed to overcome these attractive forces. Let’s dive into the key aspects to consider when comparing boiling points.
1.1 Key Factors Influencing Boiling Points
Several factors influence the boiling point of a substance. These include:
- Intermolecular Forces: These forces, which include hydrogen bonding, dipole-dipole interactions, and London dispersion forces (Van der Waals forces), play a pivotal role. Stronger forces lead to higher boiling points.
- Molecular Weight: Generally, as molecular weight increases, so does the boiling point. Larger molecules have more electrons, leading to stronger London dispersion forces.
- Molecular Shape: Molecular shape affects how molecules interact with each other. Linear molecules tend to have higher boiling points than branched molecules due to increased surface area for intermolecular interactions.
- Functional Groups: The presence of certain functional groups (such as -OH, -NH2, or -COOH) can significantly increase boiling points due to hydrogen bonding.
Understanding these factors is crucial for accurately comparing and predicting boiling points of different substances.
2. Intermolecular Forces: The Primary Determinant
Intermolecular forces are the attractive forces that exist between molecules. These forces directly impact the boiling point of a substance. The stronger the intermolecular forces, the more energy is required to separate the molecules, resulting in a higher boiling point.
2.1 Types of Intermolecular Forces
There are several types of intermolecular forces, each with varying strengths:
- Ionic Interactions: These are the strongest intermolecular forces, occurring between ions in ionic compounds. Ionic compounds typically have very high melting and boiling points.
- Hydrogen Bonding: This is a strong dipole-dipole interaction that occurs when hydrogen is bonded to highly electronegative atoms such as oxygen (O), nitrogen (N), or fluorine (F). Hydrogen bonds are stronger than typical dipole-dipole interactions and significantly increase boiling points.
- Dipole-Dipole Interactions: These occur between polar molecules that have a permanent dipole moment. The positive end of one molecule is attracted to the negative end of another.
- London Dispersion Forces (Van der Waals Forces): These are the weakest intermolecular forces and exist in all molecules, whether polar or nonpolar. They arise from temporary fluctuations in electron distribution, creating temporary dipoles.
2.2 Comparing Substances with Different Intermolecular Forces
To illustrate the impact of intermolecular forces on boiling points, let’s compare several compounds with similar molecular weights but different functional groups:
- Diethyl Ether (C4H10O): Has dipole-dipole interactions due to the polar C-O bonds. Its boiling point is 35°C.
- 1-Butanol (C4H10O): Contains a hydroxyl (-OH) group, enabling hydrogen bonding. Its boiling point is significantly higher at 117°C.
- Butane (C4H10): A nonpolar molecule with only London dispersion forces. It boils at 0°C, much lower than diethyl ether and 1-butanol.
This comparison highlights how the presence and strength of intermolecular forces dramatically influence boiling points.
2.3 The Role of Functional Groups
Functional groups play a crucial role in determining the intermolecular forces present in a molecule. Here’s how different functional groups influence boiling points:
- Alcohols (-OH): Form hydrogen bonds, leading to high boiling points.
- Carboxylic Acids (-COOH): Also form hydrogen bonds, often as dimers, further increasing boiling points.
- Amines (-NH2): Can form hydrogen bonds, though weaker than those formed by alcohols.
- Ethers (C-O-C): Exhibit dipole-dipole interactions but cannot form hydrogen bonds, resulting in lower boiling points compared to alcohols.
- Alkanes (C-H): Only have London dispersion forces, leading to the lowest boiling points among organic compounds of similar molecular weight.
For example, consider the following compounds:
- Ethanol (C2H5OH): Boiling point of 78°C due to hydrogen bonding.
- Dimethyl Ether (CH3OCH3): Boiling point of -24°C due to dipole-dipole interactions.
- Ethane (C2H6): Boiling point of -89°C due to London dispersion forces.
2.4 Ionic Compounds vs. Covalent Compounds
Ionic compounds generally have much higher boiling points than covalent compounds due to the strong electrostatic forces between ions. For instance, sodium chloride (NaCl) has a boiling point of 1413°C, while water (H2O), a covalent compound, has a boiling point of 100°C.
Table 1: Boiling Points of Different Compounds
Compound | Molecular Weight (g/mol) | Intermolecular Forces | Boiling Point (°C) |
---|---|---|---|
Butane (C4H10) | 58.12 | London Dispersion Forces | 0 |
Diethyl Ether (C4H10O) | 74.12 | Dipole-Dipole Interactions | 35 |
1-Butanol (C4H10O) | 74.12 | Hydrogen Bonding | 117 |
Sodium Butoxide (C4H9ONa) | 96.10 | Ionic Interactions | Decomposes > 260 |
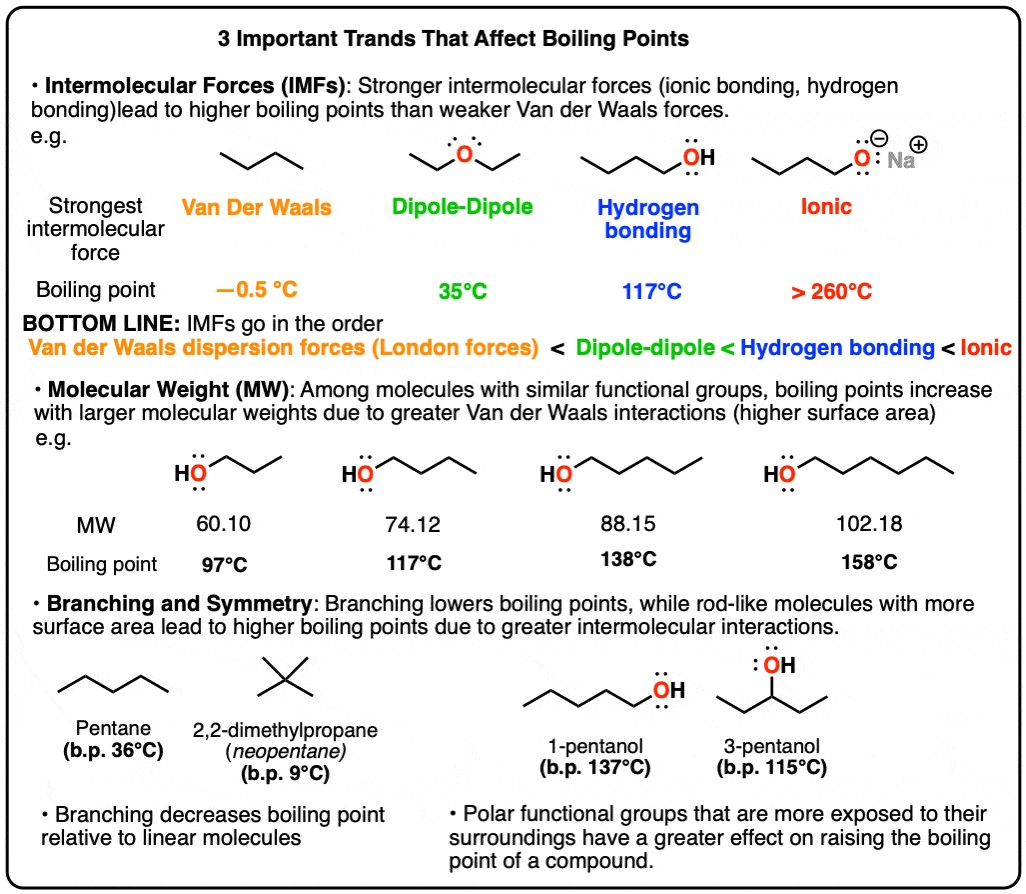
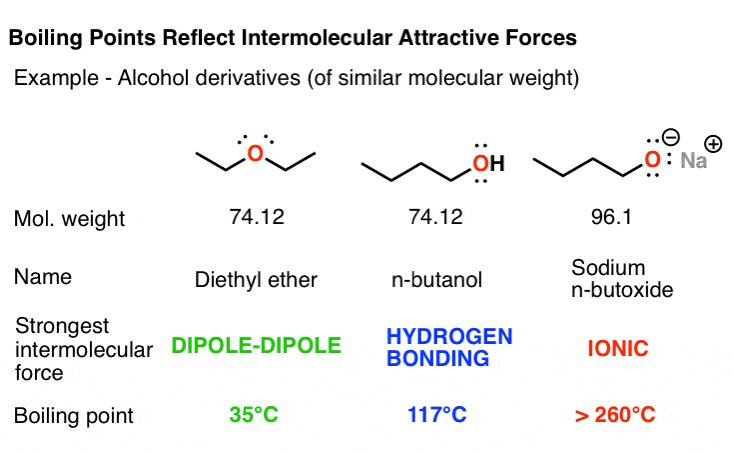
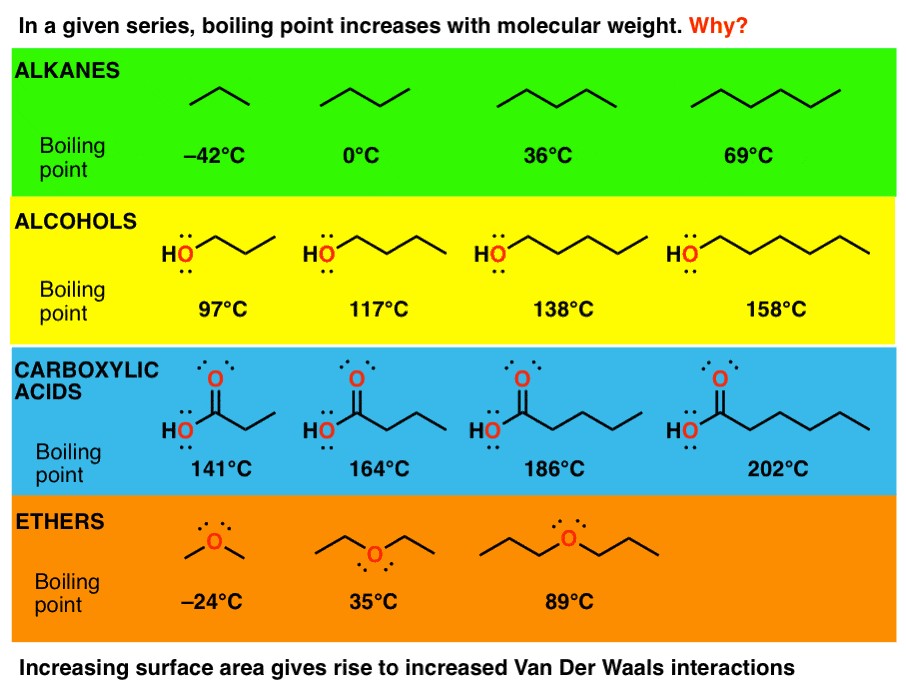
This table clearly illustrates how different intermolecular forces affect the boiling points of substances with similar molecular weights.
3. Molecular Weight: A Significant Factor
Molecular weight significantly influences boiling points, especially within a series of compounds with similar intermolecular forces. As molecular weight increases, so does the number of electrons, which enhances London dispersion forces.
3.1 The Impact of Surface Area
London dispersion forces are proportional to surface area. Longer molecules have greater surface areas, allowing for more points of contact and stronger interactions between molecules.
Consider the series of alkanes: methane (CH4), ethane (C2H6), propane (C3H8), and butane (C4H10). As the number of carbon atoms increases, the boiling point also increases:
- Methane (CH4): Boiling point of -162°C
- Ethane (C2H6): Boiling point of -89°C
- Propane (C3H8): Boiling point of -42°C
- Butane (C4H10): Boiling point of 0°C
This trend is consistent because the longer the carbon chain, the greater the surface area and the stronger the London dispersion forces.
3.2 Comparing Alkanes, Alcohols, and Carboxylic Acids
The impact of molecular weight can be seen across different classes of organic compounds.
Consider the boiling points of alkanes, alcohols, and carboxylic acids with increasing molecular weights:
Table 2: Boiling Points of Alkanes, Alcohols, and Carboxylic Acids
Compound | Molecular Weight (g/mol) | Boiling Point (°C) |
---|---|---|
Methane (CH4) | 16.04 | -162 |
Methanol (CH3OH) | 32.04 | 65 |
Ethane (C2H6) | 30.07 | -89 |
Ethanol (C2H5OH) | 46.07 | 78 |
Ethanoic Acid (CH3COOH) | 60.05 | 118 |
Propane (C3H8) | 44.10 | -42 |
Propanol (C3H7OH) | 60.10 | 97 |
Propanoic Acid (C2H5COOH) | 74.08 | 141 |
As molecular weight increases, the boiling points of each class of compound also increase. Alcohols have higher boiling points than alkanes due to hydrogen bonding, and carboxylic acids have even higher boiling points due to the ability to form strong hydrogen-bonded dimers.
3.3 The Spaghetti Analogy
A helpful analogy for understanding the effect of molecular weight on intermolecular forces is to compare molecules to strands of spaghetti. Longer strands of spaghetti are harder to pull apart because they have more surface area in contact with each other. Similarly, longer molecules have more surface area for London dispersion forces to act upon, resulting in stronger intermolecular attractions and higher boiling points.
4. Molecular Shape and Symmetry: Fine-Tuning Boiling Points
While intermolecular forces and molecular weight are primary determinants of boiling points, molecular shape and symmetry also play a significant role.
4.1 The Impact of Branching
Branched molecules tend to have lower boiling points than their linear counterparts. Branching reduces the surface area available for intermolecular interactions, weakening London dispersion forces.
Consider the isomers pentane and 2,2-dimethylpropane:
- Pentane (C5H12): A linear alkane with a boiling point of 36°C.
- 2,2-Dimethylpropane (C5H12): A highly branched alkane with a boiling point of 9°C.
The reduced boiling point of 2,2-dimethylpropane is due to its more spherical shape, which decreases the surface area available for London dispersion forces.
4.2 Symmetry and Packing
Symmetrical molecules can pack more closely together, increasing intermolecular interactions. Molecules that are rod-like in shape tend to have higher boiling points because they can align more effectively.
4.3 Alcohols: Positional Isomers
Even within a single class of compounds like alcohols, molecular shape can affect boiling points. Consider the positional isomers of pentanol:
- 1-Pentanol: The hydroxyl group is at the end of the carbon chain, making it more accessible for hydrogen bonding.
- 2-Pentanol and 3-Pentanol: The hydroxyl group is in the middle of the chain, flanked by alkyl groups, which hinder hydrogen bonding.
The boiling points decrease as the hydroxyl group becomes more hindered:
- 1-Pentanol: Higher boiling point due to more accessible hydrogen bonding.
- 3-Pentanol: Lower boiling point due to steric hindrance.
4.4 The Importance of Surface Area
The key concept underlying the influence of molecular shape on boiling points is surface area. Molecules with larger surface areas have more opportunities for intermolecular interactions, leading to higher boiling points.
The shape of a molecule affects its boiling point by influencing how well it can interact with neighboring molecules. Linear molecules generally have higher boiling points because they have a larger surface area for intermolecular attractions, whereas branched molecules have lower boiling points because their shape reduces the surface area available for interaction.
5. Boiling Points and Polarity: A Closer Look
The polarity of a molecule affects its boiling point by influencing the strength of intermolecular forces. Polar molecules have dipole-dipole interactions and, if hydrogen is bonded to oxygen, nitrogen, or fluorine, can also form hydrogen bonds, both of which increase boiling points.
5.1 Polar vs. Nonpolar Molecules
- Polar Molecules: These have an uneven distribution of electron density, resulting in a dipole moment. Examples include water (H2O) and ethanol (C2H5OH).
- Nonpolar Molecules: These have an even distribution of electron density, resulting in no net dipole moment. Examples include methane (CH4) and hexane (C6H14).
Polar molecules typically have higher boiling points than nonpolar molecules of similar molecular weight due to the presence of dipole-dipole interactions and hydrogen bonding.
5.2 Dipole-Dipole Interactions
Dipole-dipole interactions occur between polar molecules. The positive end of one molecule is attracted to the negative end of another. These interactions are stronger than London dispersion forces but weaker than hydrogen bonds.
5.3 Hydrogen Bonding
Hydrogen bonding is a special type of dipole-dipole interaction that occurs when hydrogen is bonded to highly electronegative atoms such as oxygen, nitrogen, or fluorine. Hydrogen bonds are stronger than typical dipole-dipole interactions and significantly increase boiling points.
5.4 Examples of Polarity and Boiling Point
Consider the following examples:
- Water (H2O): A polar molecule with hydrogen bonds. Boiling point of 100°C.
- Ammonia (NH3): A polar molecule with hydrogen bonds. Boiling point of -33°C.
- Ethanol (C2H5OH): A polar molecule with hydrogen bonds. Boiling point of 78°C.
- Acetone (CH3COCH3): A polar molecule with dipole-dipole interactions. Boiling point of 56°C.
- Diethyl Ether (C4H10O): A polar molecule with dipole-dipole interactions. Boiling point of 35°C.
- Hexane (C6H14): A nonpolar molecule with London dispersion forces. Boiling point of 69°C.
5.5 The Impact of Polarity on Miscibility
Polarity also affects miscibility. Polar molecules tend to be miscible with other polar molecules, while nonpolar molecules tend to be miscible with other nonpolar molecules. This principle is often summarized as “like dissolves like.”
Table 3: Polarity and Boiling Points
Compound | Polarity | Intermolecular Forces | Boiling Point (°C) |
---|---|---|---|
Water (H2O) | Polar | Hydrogen Bonds | 100 |
Ammonia (NH3) | Polar | Hydrogen Bonds | -33 |
Ethanol (C2H5OH) | Polar | Hydrogen Bonds | 78 |
Acetone (CH3COCH3) | Polar | Dipole-Dipole Interactions | 56 |
Hexane (C6H14) | Nonpolar | London Dispersion Forces | 69 |
This table shows how polarity and the resulting intermolecular forces influence the boiling points of different compounds.
6. Practical Applications: Predicting Boiling Points
Predicting boiling points involves considering all the factors discussed above: intermolecular forces, molecular weight, molecular shape, and polarity.
6.1 Step-by-Step Approach
- Identify the Functional Groups: Determine the functional groups present in the molecule, as they dictate the type of intermolecular forces.
- Assess Intermolecular Forces: Identify the primary intermolecular forces present (hydrogen bonding, dipole-dipole interactions, or London dispersion forces).
- Consider Molecular Weight: Compare the molecular weights of the compounds. Higher molecular weight generally means higher boiling point.
- Evaluate Molecular Shape: Consider the shape of the molecule (linear vs. branched) and how it affects surface area.
- Predict Relative Boiling Points: Based on these factors, predict the relative boiling points of the compounds.
6.2 Example Predictions
Example 1: Compare the boiling points of ethanol (C2H5OH) and diethyl ether (C4H10O).
- Ethanol: Has hydrogen bonding due to the -OH group.
- Diethyl Ether: Has dipole-dipole interactions due to the C-O bonds.
- Molecular Weight: Diethyl ether has a slightly higher molecular weight (74.12 g/mol) than ethanol (46.07 g/mol).
Despite the higher molecular weight of diethyl ether, ethanol has a higher boiling point (78°C) due to the stronger hydrogen bonding compared to the dipole-dipole interactions in diethyl ether (35°C).
Example 2: Compare the boiling points of pentane and hexane.
- Pentane (C5H12): Boiling point of 36°C
- Hexane (C6H14): Boiling point of 69°C
Both are nonpolar alkanes with only London dispersion forces. Hexane has a higher molecular weight and longer carbon chain, resulting in stronger London dispersion forces and a higher boiling point.
6.3 Using Boiling Point Data
Experimental boiling point data can be used to validate predictions and refine understanding of intermolecular forces. Databases and reference materials provide accurate boiling point values for a wide range of compounds.
7. Advanced Topics: Azeotropes and Pressure Dependence
Boiling points are not always straightforward, especially when dealing with mixtures or varying pressures.
7.1 Azeotropes
An azeotrope is a mixture of two or more liquids whose proportions cannot be altered by simple distillation. This occurs because, when an azeotrope is boiled, the vapor has the same proportions of constituents as the unboiled mixture. Azeotropes can have either a maximum boiling point or a minimum boiling point.
- Maximum Boiling Point Azeotropes: These have boiling points higher than any of their constituents.
- Minimum Boiling Point Azeotropes: These have boiling points lower than any of their constituents.
7.2 Pressure Dependence
Boiling points are pressure-dependent. The normal boiling point is defined as the boiling point at a pressure of 1 atmosphere (101.3 kPa). At lower pressures, the boiling point decreases, while at higher pressures, the boiling point increases.
The relationship between boiling point and pressure is described by the Clausius-Clapeyron equation:
ln(P1/P2) = -ΔHvap/R * (1/T1 - 1/T2)
Where:
- P1 and P2 are the pressures at temperatures T1 and T2, respectively.
- ΔHvap is the enthalpy of vaporization.
- R is the ideal gas constant (8.314 J/mol·K).
7.3 Vacuum Distillation
Vacuum distillation is a technique used to boil liquids at temperatures lower than their normal boiling points. This is achieved by reducing the pressure above the liquid. Vacuum distillation is particularly useful for compounds that decompose at high temperatures.
8. Real-World Examples and Applications
Understanding boiling points has numerous practical applications across various fields.
8.1 Chemical Industry
In the chemical industry, boiling points are critical for designing distillation processes to separate and purify chemical compounds. Accurate boiling point data ensures efficient and cost-effective separations.
8.2 Pharmaceutical Industry
In the pharmaceutical industry, boiling points are important for drug formulation and purification. Knowing the boiling points of solvents and active pharmaceutical ingredients (APIs) is essential for developing stable and effective drug products.
8.3 Food and Beverage Industry
In the food and beverage industry, boiling points are used in processes such as evaporation, distillation, and extraction. For example, in the production of alcoholic beverages, distillation is used to separate ethanol from water and other compounds.
8.4 Environmental Science
In environmental science, boiling points are used to identify and quantify volatile organic compounds (VOCs) in air and water samples. VOCs contribute to air pollution and can have adverse effects on human health and the environment.
8.5 Research and Development
In research and development, boiling points are used to characterize new compounds and materials. Boiling point determination is a standard analytical technique for verifying the identity and purity of synthesized compounds.
9. Common Mistakes to Avoid
When comparing boiling points, it is important to avoid common mistakes that can lead to incorrect predictions.
9.1 Neglecting Intermolecular Forces
One of the most common mistakes is neglecting the role of intermolecular forces. Intermolecular forces are the primary determinant of boiling points, and failing to consider them can lead to inaccurate predictions.
9.2 Overemphasizing Molecular Weight
While molecular weight is important, it should not be the sole factor considered. Intermolecular forces can override the effect of molecular weight, especially when comparing compounds with different functional groups.
9.3 Ignoring Molecular Shape
Molecular shape affects the surface area available for intermolecular interactions. Ignoring the shape of a molecule can lead to inaccurate predictions.
9.4 Assuming Linearity
Assuming that all molecules are linear can be misleading. Branched molecules have lower boiling points than their linear counterparts due to reduced surface area.
9.5 Overlooking Polarity
Polarity influences the strength of intermolecular forces. Overlooking polarity can lead to inaccurate predictions, especially when comparing polar and nonpolar molecules.
10. Frequently Asked Questions (FAQs)
Q1: What is the main factor that affects boiling point?
A: The primary factor is the strength of intermolecular forces between molecules.
Q2: How does molecular weight affect boiling point?
A: Generally, as molecular weight increases, boiling point also increases due to stronger London dispersion forces.
Q3: Do polar molecules have higher boiling points than nonpolar molecules?
A: Yes, polar molecules typically have higher boiling points due to dipole-dipole interactions and hydrogen bonding.
Q4: What are London dispersion forces?
A: These are weak intermolecular forces that arise from temporary fluctuations in electron distribution.
Q5: How does branching affect boiling point?
A: Branching decreases boiling point by reducing the surface area available for intermolecular interactions.
Q6: What is hydrogen bonding?
A: This is a strong dipole-dipole interaction between hydrogen and highly electronegative atoms like oxygen, nitrogen, or fluorine.
Q7: What is an azeotrope?
A: An azeotrope is a mixture of liquids that boils at a constant composition and temperature, and cannot be separated by simple distillation.
Q8: How does pressure affect boiling point?
A: Boiling point decreases with decreasing pressure and increases with increasing pressure.
Q9: Why do ionic compounds have high boiling points?
A: Ionic compounds have very strong electrostatic forces between ions, leading to high boiling points.
Q10: What is vacuum distillation?
A: It is a technique to boil liquids at lower temperatures by reducing the pressure above the liquid, useful for compounds that decompose at high temperatures.
Conclusion: Mastering Boiling Point Comparisons
Understanding how to compare boiling points requires a comprehensive grasp of intermolecular forces, molecular weight, molecular shape, and polarity. By considering these factors, you can accurately predict and compare the boiling points of different substances. For more detailed comparisons and expert analysis, visit COMPARE.EDU.VN at 333 Comparison Plaza, Choice City, CA 90210, United States or contact us via WhatsApp at +1 (626) 555-9090. COMPARE.EDU.VN is your go-to resource for making informed decisions based on thorough and reliable comparisons.
Ready to make smarter comparisons? Visit COMPARE.EDU.VN today and explore our comprehensive guides and expert insights to help you make informed decisions. Whether you’re a student, researcher, or industry professional, compare.edu.vn is here to simplify complex comparisons and empower you to choose the best options for your needs. Don’t wait – start comparing now!