Comparing the acidic strength of organic compounds can be challenging, but COMPARE.EDU.VN offers a comprehensive guide to help you understand the underlying principles and make informed assessments. Understanding the factors influencing acidity, such as periodic trends, resonance effects, and inductive effects, is crucial for predicting and comparing the behavior of organic molecules. Let’s explore these concepts in detail to master the art of evaluating acid strength and understanding acidity trends in organic molecules.
1. Understanding Acidic Strength: An Overview
1.1 What is Acidic Strength?
Acidic strength refers to the tendency of a compound to donate a proton (H+) in a solution. The stronger the acid, the greater its tendency to donate a proton. This property is quantified by the acid dissociation constant (Ka) and its logarithmic form, pKa. A lower pKa value indicates a stronger acid.
1.2 Importance of Comparing Acidic Strength
Understanding the acidic strength of organic compounds is fundamental in chemistry for several reasons:
- Predicting Reaction Outcomes: Acid-base reactions are ubiquitous in organic chemistry, and knowing the relative acidic strengths allows chemists to predict the direction and outcome of these reactions.
- Understanding Biological Processes: Many biological processes, such as enzyme catalysis, rely on precise acid-base interactions. Understanding the acidity of biomolecules helps elucidate these mechanisms.
- Designing New Molecules: In drug discovery and materials science, manipulating the acidity of molecules can alter their properties, leading to the development of new compounds with desired characteristics.
1.3 Key Factors Influencing Acidic Strength
Several factors influence the acidic strength of organic compounds, including:
- Periodic Trends: The position of the atom bearing the acidic proton on the periodic table affects its acidity.
- Resonance Effects: Delocalization of charge in the conjugate base can significantly increase acidity.
- Inductive Effects: Electron-withdrawing or electron-donating groups can influence the stability of the conjugate base and, therefore, the acidity.
- Hybridization: The hybridization state of the atom bearing the acidic proton affects its electronegativity and acidity.
- Solvent Effects: The solvent in which the acid is dissolved can influence its acidity.
2. Periodic Trends and Acidic Strength
2.1 Electronegativity and Acidity
Electronegativity plays a crucial role in determining the acidity of a compound. Electronegativity is the ability of an atom to attract electrons towards itself in a chemical bond. As electronegativity increases, the atom bearing the acidic proton can better stabilize the resulting negative charge when the proton is lost, leading to increased acidity.
2.1.1 Horizontal Trends
Moving from left to right across a row of the periodic table, electronegativity increases. This trend directly correlates with increasing acidity. Consider the following series of compounds: ethane (CH3CH3), methylamine (CH3NH2), and methanol (CH3OH).
Alternative text: Chemical structures of ethane, methylamine, and methanol, illustrating the increasing electronegativity trend from carbon to nitrogen to oxygen.
The acidity increases in the order: ethane < methylamine < methanol. This is because oxygen is more electronegative than nitrogen, which is more electronegative than carbon. The conjugate base of methanol (methoxide) is more stable than the conjugate base of methylamine, which is more stable than the conjugate base of ethane. The more stable the conjugate base, the stronger the acid.
2.1.2 Vertical Trends
Moving down a group of the periodic table, electronegativity generally decreases, but the atomic size increases significantly. The size effect often outweighs the electronegativity effect in determining acidity.
Consider the haloacids: HF, HCl, HBr, and HI.
Alternative text: Representation of haloacids HF, HCl, HBr, and HI, showing the increasing atomic size of the halogen atoms down the group.
Acidity increases in the order: HF < HCl < HBr < HI. Although fluorine is the most electronegative halogen, the fluoride ion is the least stable because its small size concentrates the negative charge in a small volume. As we move down the group, the size of the halide ions increases, spreading the negative charge over a larger volume and stabilizing the ion. This charge delocalization makes the larger halide ions more stable, leading to increased acidity of the corresponding haloacids.
2.2 Atomic Size and Charge Delocalization
As mentioned earlier, the size of the atom bearing the negative charge in the conjugate base plays a critical role. Larger atoms can better stabilize the negative charge because the charge is spread out over a larger volume.
Alternative text: Illustration of charge distribution in halide ions, demonstrating how larger ions (like iodide) spread the negative charge over a larger volume, increasing stability.
This concept is crucial for understanding the acidity of thiols (RSH) compared to alcohols (ROH). Sulfur is larger than oxygen, so thiols are generally more acidic than alcohols. For example, the pKa of the thiol group in cysteine is approximately 8.3, while the pKa of the hydroxyl group in serine is around 17.
2.3 Periodic Trends Summary
Trend | Direction | Effect on Acidity | Reason |
---|---|---|---|
Electronegativity | Left to Right (Periodic Table) | Increases | More electronegative atoms better stabilize negative charge. |
Atomic Size | Top to Bottom (Periodic Table) | Increases | Larger atoms allow for greater charge delocalization and stabilization. |
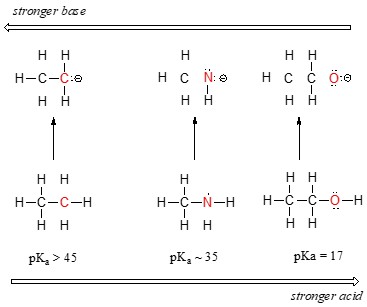
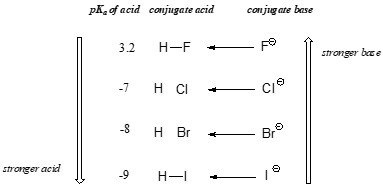
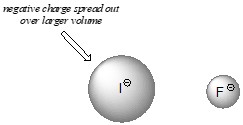
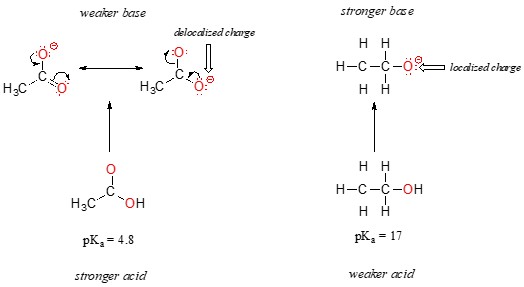
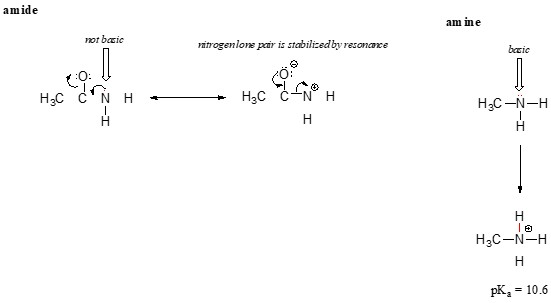
3. Resonance Effects on Acidic Strength
3.1 Introduction to Resonance
Resonance occurs when a molecule can be represented by two or more Lewis structures that differ only in the distribution of electrons. The actual structure of the molecule is a hybrid of these resonance structures, and the electrons are said to be delocalized. Resonance delocalization can significantly stabilize a conjugate base, leading to increased acidity.
3.2 Acidity of Carboxylic Acids vs. Alcohols
Carboxylic acids (RCOOH) are much more acidic than alcohols (ROH) due to resonance stabilization of the carboxylate anion (RCOO-).
Alternative text: Comparison of ethoxide and acetate ions, illustrating resonance delocalization of the negative charge in the acetate ion, making it more stable.
In the carboxylate anion, the negative charge is delocalized over both oxygen atoms, resulting in a more stable conjugate base and a stronger acid. In contrast, the negative charge in the alkoxide ion is localized on a single oxygen atom, making it less stable. The pKa of acetic acid is around 4.76, while the pKa of ethanol is around 16, demonstrating the significant impact of resonance on acidity.
3.3 Amides vs. Amines
Resonance also explains why nitrogen atoms in amides (RCONH2) are much less basic than nitrogen atoms in amines (RNH2). In amides, the lone pair of electrons on the nitrogen atom is delocalized into the carbonyl group through resonance.
Alternative text: Resonance structures of an amide, showing the delocalization of the nitrogen lone pair into the carbonyl group, reducing its basicity.
This delocalization makes the lone pair less available for bonding with a proton, thus decreasing the basicity of the nitrogen atom. Amines, lacking this resonance stabilization, are much more basic.
3.4 Identifying the Most Acidic Proton
In molecules with multiple potential acidic protons, resonance effects can help identify the most acidic one. Consider ascorbic acid (Vitamin C), which has a pKa of 4.1.
Alternative text: Structure of ascorbic acid (Vitamin C), highlighting the hydroxyl group whose deprotonation leads to resonance stabilization of the resulting anion.
The most acidic proton is the one whose removal results in a conjugate base with the greatest resonance stabilization. In ascorbic acid, deprotonation of the hydroxyl group at the 3-position leads to a conjugate base in which the negative charge can be delocalized over two oxygen atoms, making it the most acidic proton.
3.5 Resonance Effects Summary
Factor | Description | Effect on Acidity/Basicity | Example |
---|---|---|---|
Resonance | Delocalization of electrons through multiple resonance structures. | Increases acidity by stabilizing the conjugate base. Decreases basicity by making lone pairs less available. | Carboxylic acids vs. alcohols, amides vs. amines |
4. Inductive Effects on Acidic Strength
4.1 Introduction to Inductive Effects
Inductive effects arise from the unequal sharing of electrons in a sigma bond due to differences in electronegativity between atoms. Electronegative atoms or groups can withdraw electron density through sigma bonds (electron-withdrawing groups, or EWGs), while electropositive atoms or groups can donate electron density (electron-donating groups, or EDGs).
4.2 Electron-Withdrawing Groups (EWGs)
EWGs stabilize the conjugate base by pulling electron density away from the negatively charged atom, thus increasing acidity. The more EWGs present and the closer they are to the acidic proton, the stronger the acid.
Consider the series of chlorinated acetic acids: acetic acid, monochloroacetic acid, dichloroacetic acid, and trichloroacetic acid.
Alternative text: Structures of acetic acid and its chlorinated derivatives, showing the increasing electron-withdrawing effect of chlorine atoms, leading to increased acidity.
The pKa values decrease as the number of chlorine atoms increases:
- Acetic acid: pKa = 4.76
- Monochloroacetic acid: pKa = 2.87
- Dichloroacetic acid: pKa = 1.48
- Trichloroacetic acid: pKa = 0.70
Each chlorine atom withdraws electron density, stabilizing the carboxylate anion and increasing acidity.
4.3 Electron-Donating Groups (EDGs)
EDGs destabilize the conjugate base by increasing the electron density on the negatively charged atom, thus decreasing acidity. The more EDGs present and the closer they are to the acidic proton, the weaker the acid.
Consider the acidity of alcohols with different alkyl substituents. Bulky alkyl groups are electron-donating and destabilize the conjugate base, making the alcohol less acidic.
4.4 Distance Dependence
The inductive effect decreases with distance. An EWG or EDG closer to the acidic proton has a greater effect than one farther away. For example, 2-chlorobutanoic acid is more acidic than 4-chlorobutanoic acid.
4.5 Comparing Inductive and Resonance Effects
Resonance effects are generally more powerful than inductive effects. While inductive effects can fine-tune acidity, resonance effects can cause much larger differences in acidic strength. For example, the difference in acidity between an alcohol and a carboxylic acid is primarily due to resonance stabilization, which far outweighs any inductive effects.
4.6 Inductive Effects Summary
Factor | Description | Effect on Acidity | Example |
---|---|---|---|
Electron-Withdrawing Group | Atoms or groups that pull electron density through sigma bonds. | Increases acidity by stabilizing the conjugate base. | Chlorinated acetic acids |
Electron-Donating Group | Atoms or groups that donate electron density through sigma bonds. | Decreases acidity by destabilizing the conjugate base. | Alcohols with bulky alkyl substituents |
Distance Dependence | The inductive effect decreases with distance from the acidic proton. | EWGs or EDGs closer to the acidic proton have a greater effect than those farther away. | Comparing 2-chlorobutanoic acid with 4-chlorobutanoic acid |
5. Hybridization and Acidic Strength
5.1 Introduction to Hybridization
The hybridization state of the carbon atom bearing the acidic proton also influences acidity. Carbon atoms can be sp, sp2, or sp3 hybridized, and the acidity of a C-H bond increases with increasing s-character in the hybrid orbital.
5.2 S-Character and Electronegativity
Higher s-character means that the electrons in the bond are held closer to the nucleus, making the carbon atom effectively more electronegative. This increased electronegativity stabilizes the conjugate base, leading to increased acidity.
5.3 Comparing Alkynes, Alkenes, and Alkanes
Consider the following series:
- Alkynes (sp hybridization): C≡C-H
- Alkenes (sp2 hybridization): C=C-H
- Alkanes (sp3 hybridization): C-C-H
The acidity increases in the order: alkanes < alkenes < alkynes.
Alkynes have the highest s-character (50%), followed by alkenes (33%), and then alkanes (25%). The sp hybridized carbon in alkynes is more electronegative than the sp2 hybridized carbon in alkenes or the sp3 hybridized carbon in alkanes, making alkynes the most acidic.
5.4 Hybridization Effects Summary
Factor | Description | Effect on Acidity | Example |
---|---|---|---|
Hybridization | The state of hybridization of the carbon atom bearing the acidic proton (sp, sp2, or sp3). | Acidity increases with increasing s-character (sp > sp2 > sp3). | Comparing alkynes, alkenes, and alkanes |
6. Solvent Effects on Acidic Strength
6.1 Introduction to Solvent Effects
The solvent in which an acid is dissolved can significantly influence its acidity. Solvents can stabilize the acid, the conjugate base, or both, thereby affecting the equilibrium of the acid dissociation reaction.
6.2 Protic vs. Aprotic Solvents
- Protic Solvents: Protic solvents (e.g., water, alcohols) can donate hydrogen bonds. They can stabilize both the acid and the conjugate base through solvation, but they typically stabilize the conjugate base more effectively, increasing acidity.
- Aprotic Solvents: Aprotic solvents (e.g., DMSO, DMF) cannot donate hydrogen bonds. They primarily solvate cations and have a smaller effect on the solvation of anions (conjugate bases). In aprotic solvents, the intrinsic acidity of the acid is more pronounced because the conjugate base is less stabilized.
6.3 Leveling Effect
The leveling effect refers to the phenomenon where the acidity of strong acids is “leveled” to the acidity of the hydronium ion (H3O+) in water. Strong acids that are stronger than H3O+ will completely dissociate in water, and their acidity will be indistinguishable. Similarly, strong bases that are stronger than hydroxide (OH-) will be leveled to the basicity of hydroxide.
6.4 Solvent Effects Summary
Factor | Description | Effect on Acidity | Example |
---|---|---|---|
Protic Solvent | Solvents that can donate hydrogen bonds (e.g., water, alcohols). | Stabilize both acid and conjugate base, typically increasing acidity. | Acidity of haloacids in water |
Aprotic Solvent | Solvents that cannot donate hydrogen bonds (e.g., DMSO, DMF). | Primarily solvate cations, leading to a more pronounced intrinsic acidity. | Acidity of carboxylic acids in DMSO |
Leveling Effect | The acidity of strong acids is leveled to the acidity of the hydronium ion in water. | Strong acids stronger than H3O+ will completely dissociate, and their acidity is the same. | Comparing HCl and H2SO4 in water |
7. Putting It All Together: Examples and Practice
7.1 Ranking Acidic Strength
To rank the acidic strength of organic compounds, consider the following steps:
- Identify the Acidic Proton: Determine which proton is most likely to be donated.
- Draw the Conjugate Base: Draw the structure of the conjugate base formed after the proton is removed.
- Assess Stability Factors: Evaluate the stability of the conjugate base by considering:
- Periodic Trends: Electronegativity and atomic size.
- Resonance Effects: Delocalization of charge.
- Inductive Effects: Presence of EWGs or EDGs.
- Hybridization: s-character of the carbon atom.
- Solvent Effects: Type of solvent and its ability to solvate ions.
- Rank Acidity: The more stable the conjugate base, the stronger the acid.
7.2 Example 1: Comparing Phenol and Ethanol
Which is more acidic: phenol or ethanol?
Alternative text: Chemical structures of phenol and ethanol, demonstrating the resonance stabilization of the phenoxide ion compared to the ethoxide ion.
- Phenol: The conjugate base, phenoxide, is stabilized by resonance delocalization of the negative charge into the aromatic ring.
- Ethanol: The conjugate base, ethoxide, has no resonance stabilization and the negative charge is localized on the oxygen atom.
Therefore, phenol is much more acidic than ethanol due to the resonance stabilization of the phenoxide ion.
7.3 Example 2: Comparing Acetic Acid and Formic Acid
Which is more acidic: acetic acid (CH3COOH) or formic acid (HCOOH)?
- Acetic Acid: The methyl group (CH3) is a weak electron-donating group, which slightly destabilizes the conjugate base.
- Formic Acid: Has no electron-donating groups.
Therefore, formic acid is slightly more acidic than acetic acid because the electron-donating methyl group in acetic acid destabilizes the conjugate base.
7.4 Practice Problems
-
Rank the following compounds in order of increasing acidity:
- Methane (CH4)
- Ammonia (NH3)
- Water (H2O)
- Hydrogen Fluoride (HF)
-
Which is more acidic: benzoic acid or p-methoxybenzoic acid? Explain your reasoning.
-
Explain why trifluoroacetic acid (CF3COOH) is a stronger acid than acetic acid (CH3COOH).
8. Conclusion: Mastering Acidic Strength Comparisons
Understanding and comparing the acidic strength of organic compounds is a fundamental skill in chemistry. By considering periodic trends, resonance effects, inductive effects, hybridization, and solvent effects, you can predict and explain the relative acidity of different molecules. This knowledge is essential for understanding chemical reactions, biological processes, and for designing new molecules with desired properties.
Are you ready to dive deeper and explore more comparisons? Visit COMPARE.EDU.VN to discover comprehensive guides and resources that will help you make informed decisions and gain a competitive edge.
9. Frequently Asked Questions (FAQ)
-
What is pKa and how does it relate to acidic strength?
- pKa is the negative logarithm of the acid dissociation constant (Ka). A lower pKa value indicates a stronger acid, meaning it more readily donates a proton.
-
Why are carboxylic acids more acidic than alcohols?
- Carboxylic acids are more acidic than alcohols due to resonance stabilization of the carboxylate anion, which delocalizes the negative charge over two oxygen atoms.
-
How do electron-withdrawing groups affect acidity?
- Electron-withdrawing groups increase acidity by stabilizing the conjugate base. They pull electron density away from the negatively charged atom, making the conjugate base more stable.
-
Do electron-donating groups increase or decrease acidity?
- Electron-donating groups decrease acidity by destabilizing the conjugate base. They increase the electron density on the negatively charged atom, making the conjugate base less stable.
-
What is the role of atomic size in determining acidity?
- Larger atoms can better stabilize a negative charge because the charge is spread out over a larger volume. This effect is particularly important when comparing elements within the same group of the periodic table.
-
How does hybridization affect the acidity of C-H bonds?
- The acidity of a C-H bond increases with increasing s-character in the hybrid orbital. Thus, alkynes (sp) are more acidic than alkenes (sp2), which are more acidic than alkanes (sp3).
-
What are protic and aprotic solvents and how do they affect acidity?
- Protic solvents can donate hydrogen bonds and typically stabilize both the acid and the conjugate base, increasing acidity. Aprotic solvents cannot donate hydrogen bonds and primarily solvate cations, leading to a more pronounced intrinsic acidity.
-
What is the leveling effect?
- The leveling effect is the phenomenon where the acidity of strong acids is “leveled” to the acidity of the hydronium ion (H3O+) in water. Strong acids that are stronger than H3O+ will completely dissociate in water, and their acidity will be indistinguishable.
-
How can I predict the most acidic proton in a molecule with multiple functional groups?
- To predict the most acidic proton, draw the conjugate base for each potential acidic proton and assess the stability of each conjugate base considering factors such as resonance, inductive effects, and atomic size.
-
Where can I find more information and comparisons on organic compounds?
- Visit COMPARE.EDU.VN for comprehensive guides, comparisons, and resources to help you make informed decisions and expand your knowledge of organic chemistry.
10. Explore More at COMPARE.EDU.VN
Ready to make informed decisions? Visit COMPARE.EDU.VN today to explore in-depth comparisons and resources designed to simplify your choices.
For further assistance, contact us:
Address: 333 Comparison Plaza, Choice City, CA 90210, United States
Whatsapp: +1 (626) 555-9090
Website: COMPARE.EDU.VN
Let compare.edu.vn be your guide to clarity and confidence in every comparison!