Unraveling the complexities of quantum computing requires understanding its fundamental building blocks. Can You Compare Uip Cip? This article, brought to you by COMPARE.EDU.VN, delves into the innovative world of Microsoft’s Majorana 1 quantum chip and its Topological Core architecture, comparing it to other approaches and highlighting its potential to revolutionize industries. Explore the difference between topological qubits and conventional qubits.
1. Understanding the Basics: Quantum Computing and Qubits
1.1. The Quantum Leap in Computation
Quantum computing represents a paradigm shift from classical computing, leveraging the principles of quantum mechanics to solve problems that are intractable for even the most powerful supercomputers. While classical computers use bits that can be either 0 or 1, quantum computers use qubits. Qubits can exist in a superposition of both 0 and 1 simultaneously, enabling them to perform complex calculations much more efficiently. This opens up possibilities in fields like drug discovery, materials science, and cryptography. Quantum computing has been around for a bit but it’s starting to ramp up exponentially.
1.2. The Challenge of Qubit Stability
Qubits are notoriously susceptible to environmental noise and perturbations, leading to errors in computation. This “decoherence” is a major hurdle in building practical quantum computers. Maintaining the stability and coherence of qubits is a critical focus of quantum research. The creation of the first Majorana 1 Chip will have a big step in determining the path to the future.
1.3. Comparing Qubit Types: A Brief Overview
Different types of qubits exist, each with its own strengths and weaknesses. Superconducting qubits, trapped ion qubits, and topological qubits are among the most prominent. Topological qubits, like those used in Microsoft’s Majorana 1 chip, are designed to be inherently more stable and resistant to errors. To fully understand this new system, we will compare Uip Cip and other systems.
2. Introducing Microsoft’s Majorana 1 and Topological Qubits
2.1. A Breakthrough in Quantum Architecture
Microsoft’s introduction of the Majorana 1 quantum chip marks a significant milestone in quantum computing. Powered by a new Topological Core architecture, it aims to create quantum computers capable of tackling complex industrial-scale problems. This new architecture has been developed to fit a million qubits on a single chip, and should fit in the palm of one’s hand, according to Microsoft.
2.2. What Makes Topological Qubits Different?
Topological qubits offer a unique approach to quantum computing by encoding information in the “topology” of the qubit, rather than the specific state of a particle. This makes them inherently more resistant to environmental noise and errors.
2.3. The Role of Topoconductors
The Majorana 1 chip leverages topoconductors, a new type of material that can observe and control Majorana particles. Majorana particles are their own antiparticles and exhibit unique quantum properties that contribute to the stability and scalability of qubits.
3. Key Features and Advantages of the Majorana 1 Chip
3.1. Error Resistance at the Hardware Level
The Topological Core architecture incorporates error resistance directly into the hardware, making the Majorana 1 chip more stable and reliable. This reduces the need for complex error correction algorithms, simplifying the quantum computing process.
3.2. Scalability to a Million Qubits
Microsoft’s architecture offers a clear path to fitting a million qubits on a single chip, a critical threshold for quantum computers to deliver transformative solutions. This scalability is essential for tackling complex industrial and societal problems.
3.3. Digital Control of Qubits
The Majorana 1 chip enables qubits to be controlled digitally, simplifying how quantum computing works and reducing the reliance on fine-tuned analog control. This digital control simplifies the quantum computing process and the physical requirements to build a scalable machine.
4. Comparing Majorana 1 with Other Quantum Computing Approaches
4.1. Superconducting Qubits: A Comparison
Superconducting qubits are a leading approach in quantum computing, but they are highly susceptible to noise and require complex error correction. Majorana 1’s topological qubits offer inherent error resistance, potentially reducing the overhead associated with error correction.
4.2. Trapped Ion Qubits: A Contrast
Trapped ion qubits offer high fidelity but can be challenging to scale. Majorana 1’s architecture aims to provide both stability and scalability, addressing a key limitation of trapped ion qubits.
4.3. Photonic Qubits: A Different Paradigm
Photonic qubits use photons as qubits, offering advantages in terms of coherence and connectivity. However, they can be difficult to control and entangle. Majorana 1’s digital control and error resistance provide a different set of advantages.
5. Potential Applications of Million-Qubit Quantum Computers
5.1. Revolutionizing Materials Science
Million-qubit quantum computers can simulate the behavior of molecules and materials with unprecedented accuracy, leading to the discovery of new materials with desired properties. This could revolutionize industries ranging from construction to healthcare.
5.2. Solving Complex Chemistry Problems
Quantum computers can tackle challenging chemistry problems, such as understanding why materials suffer corrosion or cracks. This could lead to self-healing materials and more durable products.
5.3. Catalysis and Pollution Reduction
Quantum computing can help design catalysts to break down pollutants into valuable byproducts, offering solutions for cleaning up microplastics and tackling carbon pollution. This could lead to more sustainable industrial processes.
5.4. Advancements in Healthcare and Agriculture
Quantum computing can enable more effective use of enzymes in healthcare and agriculture, leading to breakthroughs in eradicating global hunger and improving healthcare outcomes.
6. The Science Behind Topological Qubits and Majoranas
6.1. Understanding Topological States of Matter
Topological qubits rely on topological states of matter, which exhibit unique quantum properties that make them resistant to local perturbations. This robustness is key to the stability of topological qubits.
6.2. The Nature of Majorana Particles
Majorana particles are their own antiparticles and exist at the edges of topological materials. Their unique quantum properties make them ideal for encoding and manipulating quantum information in a robust manner.
6.3. Creating and Measuring Majorana Particles
Microsoft researchers have developed a new materials stack made of indium arsenide and aluminum to create Majorana particles and accurately measure their properties. This breakthrough is essential for practical quantum computing.
7. Microsoft’s Path to Scalable Quantum Computing
7.1. Overcoming Scientific and Engineering Challenges
Microsoft has invested years in overcoming the scientific and engineering challenges associated with topological qubits, including developing new materials and measurement techniques.
7.2. The DARPA US2QC Program
Microsoft is one of two companies invited to the final phase of DARPA’s Underexplored Systems for Utility-Scale Quantum Computing (US2QC) program, which aims to deliver the industry’s first utility-scale fault-tolerant quantum computer.
7.3. Partnerships with Quantinuum and Atom Computing
Microsoft has partnered with Quantinuum and Atom Computing to reach scientific and engineering breakthroughs with today’s qubits, demonstrating the potential of hybrid quantum systems.
8. The Quantum Ecosystem: Hardware, Software, and AI
8.1. Integrating Quantum Hardware with Classical Computing
Quantum computers will not replace classical computers but will work alongside them to solve complex problems. Integrating quantum hardware with classical computing resources is essential for practical applications.
8.2. The Role of AI in Quantum Computing
AI can play a crucial role in optimizing quantum algorithms, controlling qubits, and analyzing quantum data. The combination of AI and quantum computing has the potential to accelerate scientific discovery and innovation.
8.3. Azure Quantum: A Suite of Integrated Solutions
Azure Quantum offers a suite of integrated solutions that allow customers to leverage leading AI, high-performance computing, and quantum platforms in Azure to advance scientific discovery.
9. Rethinking Quantum Computing at Scale
9.1. The Need for a Million Qubits
Reaching the next horizon of quantum computing requires a quantum architecture that can provide a million qubits or more and reach trillions of fast and reliable operations.
9.2. Designing Quantum Materials Atom by Atom
Microsoft’s topological qubit architecture involves designing quantum materials atom by atom, ensuring that the materials line up perfectly to create stable qubits.
9.3. The Majorana 1 Chip: A Step Towards Scalability
The Majorana 1 chip is a significant step towards scalable quantum computing, demonstrating the potential of topological qubits to overcome the limitations of other approaches.
10. Real-World Impact and Future Outlook
10.1. Transforming Industries with Quantum Computing
Quantum computing has the potential to transform industries by enabling the design of new materials, the discovery of new drugs, and the optimization of complex systems.
10.2. The Quantum Ready Program
Microsoft’s Quantum Ready program aims to prepare individuals and organizations for the quantum future by providing training, tools, and resources.
10.3. The Future of Quantum Computing
The future of quantum computing is bright, with ongoing research and development efforts focused on improving qubit stability, scalability, and control. Quantum computing promises to revolutionize various fields and create new opportunities for innovation.
11. Detailed Comparison Table: Topological vs. Other Qubit Technologies
Feature | Topological Qubits (Majorana 1) | Superconducting Qubits | Trapped Ion Qubits | Photonic Qubits |
---|---|---|---|---|
Stability | High (Error Resistance) | Low (Requires Error Correction) | Medium | High |
Scalability | High (Path to Million Qubits) | Medium | Low | Medium |
Control | Digital (Simplified) | Analog (Fine-Tuned) | Analog | Complex |
Coherence Time | Long | Medium | Long | Long |
Error Correction | Less Required | High Requirement | Medium Requirement | Medium Requirement |
Materials | Indium Arsenide, Aluminum | Aluminum, Niobium | Ions | Photons |
Applications | Materials Science, Chemistry | General Purpose | Precision Computing | Communication |
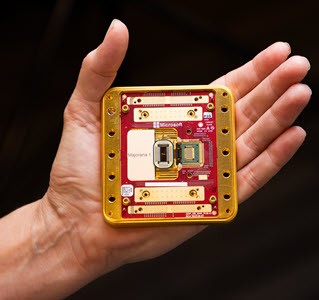
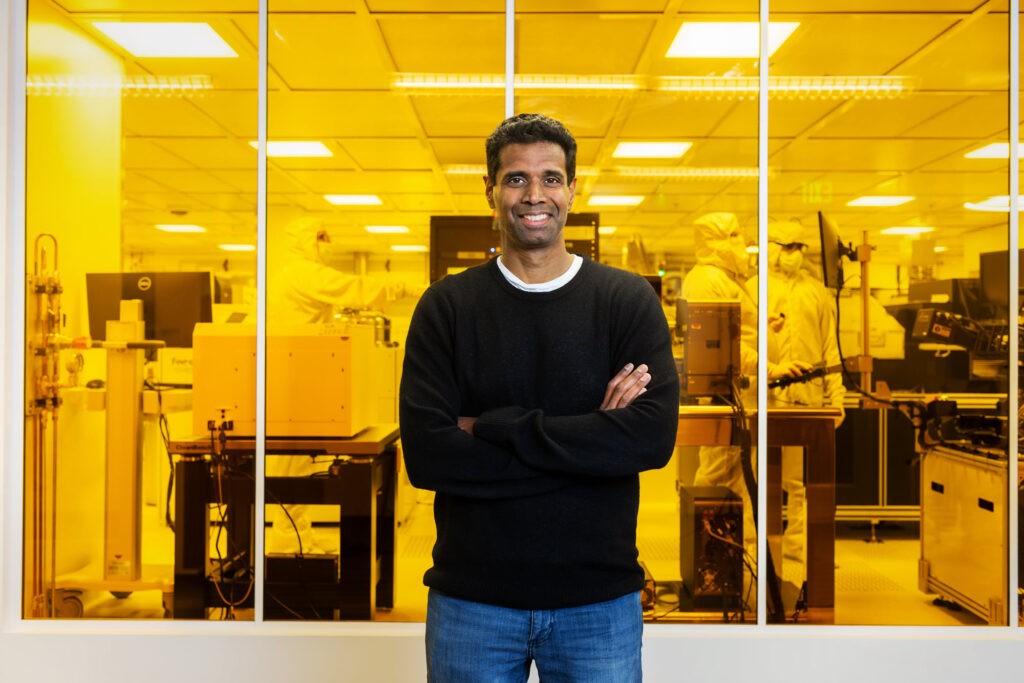
12. Understanding UIP (Uninterruptible Power) and CIP (Clean-In-Place)
12.1. UIP in Industrial Applications
Uninterruptible Power (UIP) refers to systems designed to provide continuous power supply, even during power outages or fluctuations. These systems are critical in industries where downtime can lead to significant losses or safety hazards. In quantum computing, a stable power supply is crucial for maintaining the delicate quantum states of qubits.
12.2. CIP in Manufacturing
Clean-In-Place (CIP) is a method of cleaning interior surfaces of pipes, vessels, process equipment, filters, and associated fittings, without disassembly. CIP systems are essential in industries such as food processing, pharmaceuticals, and biotechnology, where hygiene and sterility are paramount.
12.3. Relevance to Quantum Computing
While UIP and CIP are not directly related to the core technology of quantum computing, they are important considerations for the infrastructure supporting quantum computers. A reliable power supply (UIP) ensures that the quantum computer can operate continuously, while clean manufacturing processes (CIP) are necessary for producing the high-purity materials and components used in quantum devices.
13. Comparing Quantum Computing Companies
13.1. Microsoft’s Approach
Microsoft focuses on topological qubits for inherent stability and scalability, aiming for practical, commercially viable quantum computers.
13.2. Google’s Approach
Google uses superconducting qubits, emphasizing quantum supremacy and near-term applications with high qubit counts.
13.3. IBM’s Approach
IBM also utilizes superconducting qubits, focusing on developing a full-stack quantum computing platform for enterprise use.
13.4. Other Players
Other companies like Rigetti, IonQ, and Quantum Circuits Inc. are exploring different qubit technologies and quantum computing architectures.
14. The Importance of Error Correction in Quantum Computing
14.1. The Challenge of Decoherence
Decoherence, the loss of quantum information due to environmental noise, is a major obstacle in quantum computing. Error correction techniques are essential for mitigating the effects of decoherence.
14.2. Topological Qubits and Error Resistance
Topological qubits offer inherent error resistance by encoding information in the topology of the qubit, reducing the need for complex error correction algorithms.
14.3. Quantum Error Correction Codes
Quantum error correction codes are used to detect and correct errors in quantum computations, enabling fault-tolerant quantum computing.
15. FAQs About Quantum Computing and Majorana 1
15.1. What is quantum computing?
Quantum computing is a new paradigm of computation that leverages the principles of quantum mechanics to solve complex problems.
15.2. How does quantum computing differ from classical computing?
Quantum computing uses qubits that can exist in a superposition of both 0 and 1 simultaneously, enabling them to perform complex calculations much more efficiently than classical computers.
15.3. What are qubits?
Qubits are the basic units of information in quantum computing, analogous to bits in classical computing.
15.4. What are topological qubits?
Topological qubits are a type of qubit that encodes information in the topology of the qubit, making them inherently more resistant to environmental noise and errors.
15.5. What is Majorana 1?
Majorana 1 is Microsoft’s quantum chip powered by a new Topological Core architecture, designed to create quantum computers capable of tackling complex industrial-scale problems.
15.6. What are topoconductors?
Topoconductors are a new type of material that can observe and control Majorana particles, contributing to the stability and scalability of qubits.
15.7. What are Majorana particles?
Majorana particles are their own antiparticles and exhibit unique quantum properties that contribute to the stability and scalability of qubits.
15.8. What are the potential applications of quantum computing?
Quantum computing has potential applications in materials science, chemistry, drug discovery, cryptography, and more.
15.9. How does Microsoft’s approach to quantum computing differ from other companies?
Microsoft focuses on topological qubits for inherent stability and scalability, while other companies use different qubit technologies and architectures.
15.10. How can I learn more about quantum computing?
You can explore online courses, research papers, and resources like Microsoft’s Quantum Ready program to learn more about quantum computing.
16. Conclusion: The Quantum Horizon
Microsoft’s Majorana 1 chip represents a significant step towards scalable and reliable quantum computing. By leveraging topological qubits and innovative materials, Microsoft aims to unlock the full potential of quantum computing and transform industries. While challenges remain, the progress made with Majorana 1 demonstrates the promise of quantum computing to solve some of the world’s most complex problems.
Quantum computing is advancing rapidly, and COMPARE.EDU.VN is committed to providing you with the latest insights and comparisons to help you understand this transformative technology.
Ready to explore more comparisons and make informed decisions? Visit COMPARE.EDU.VN today and unlock the power of knowledge. Our comprehensive resources are designed to help you navigate the complexities of technology, education, and more.
Contact us:
Address: 333 Comparison Plaza, Choice City, CA 90210, United States
Whatsapp: +1 (626) 555-9090
Website: compare.edu.vn