A hydrogen bond stands out as a unique intermolecular force (IMF), a special type of dipole-dipole attraction that occurs when a hydrogen atom, linked to a highly electronegative atom, finds itself close to another electronegative atom boasting lone pair electrons. These intermolecular forces are the interactions that happen between molecules, distinct from the bonds within them, and include common dipole-dipole interactions and dispersion forces. Hydrogen bonds generally assert themselves as stronger than typical dipole-dipole and dispersion forces, yet remain weaker than the robust covalent and ionic bonds.
Unveiling Hydrogen Bonding Through Boiling Points
Many elements can form compounds with hydrogen. When examining the boiling points of hydrogen compounds within group 14 of the periodic table, a clear trend emerges: boiling points ascend as you move down the group.
Figure 1: Boiling point trend of group 14 element hydrides, illustrating the impact of increasing molecular size and dispersion forces.
This increase is attributed to the growing molecular size and the greater number of electrons, which amplify van der Waals dispersion forces. However, when we shift our focus to the hydrogen compounds of elements in groups 15, 16, and 17, an anomaly appears.
Figure 2: Boiling points of hydrides in groups 15-17, demonstrating unexpectedly high boiling points for NH3, H2O, and HF due to hydrogen bonding.
While the trend observed in group 14 might suggest a similar pattern for groups 15-17, the boiling points of the hydrogen compound of the first element in each of these groups—nitrogen (N), oxygen (O), and fluorine (F)—are unexpectedly high. Ammonia (NH3), water (H2O), and hydrogen fluoride (HF) exhibit significantly elevated boiling points compared to what would be predicted based on their group trends. This deviation indicates the presence of additional, stronger intermolecular forces requiring more thermal energy to overcome. These potent forces are what we define as hydrogen bonds. By comparing boiling points, we can infer the presence and relative strength of hydrogen bonding in these compounds. The higher boiling points of (NH_3), (H_2O), and (HF) directly demonstrate the substantial energy needed to break these hydrogen bonds, in contrast to weaker intermolecular forces in similar molecules without hydrogen bonding.
The Molecular Origins of Hydrogen Bond Strength
The capacity for hydrogen bonding is not universal; it’s specific to molecules containing hydrogen bonded to highly electronegative atoms such as nitrogen, oxygen, or fluorine.
Figure 3: Molecular structures of ammonia, water, and hydrogen fluoride, highlighting the crucial lone pairs on electronegative atoms that facilitate hydrogen bonding.
Let’s examine the key characteristics of molecules capable of hydrogen bonding, using (NH_3), (H_2O), and (HF) as examples:
- Hydrogen atom attached to a highly electronegative atom: This creates a significant positive partial charge ((delta+)) on the hydrogen atom. The high electronegativity of nitrogen, oxygen, and fluorine pulls electron density away from the hydrogen, making it highly positive.
- Highly electronegative atom with at least one “active” lone pair: These electronegative atoms (N, O, F) also carry a significant negative partial charge ((delta-)) and possess lone pairs of electrons. Lone pairs in the second energy level (n=2) are particularly effective due to their compactness, leading to a high negative charge density and a strong attraction for positive charges. Lone pairs at higher energy levels are more diffuse, resulting in lower charge density and weaker attraction.
To understand how hydrogen bonds affect boiling points, consider water molecules approaching each other.
Figure 4: Depiction of hydrogen bonding between water molecules, illustrating the strong dipole-dipole interactions.
The (delta+) hydrogen atom of one water molecule is strongly attracted to the lone pair of electrons on the oxygen atom of a neighboring water molecule. This attraction is substantial, almost resembling the initial stages of forming a coordinate (dative covalent) bond. Although not as strong as a covalent bond, this hydrogen bond is significantly more potent than ordinary dipole-dipole interactions. Hydrogen bonds possess about one-tenth the strength of a typical covalent bond and are continuously forming and breaking in liquid water.
Water serves as an excellent example for illustrating how hydrogen bonding elevates boiling points. Each water molecule can potentially form four hydrogen bonds with surrounding water molecules: two through its hydrogen atoms and two through its oxygen atom’s lone pairs. This optimal arrangement, where every hydrogen and lone pair can participate in hydrogen bonding, leads to a robust network.
This extensive hydrogen bonding network is the primary reason why water’s boiling point is higher than that of ammonia or hydrogen fluoride. In ammonia, each nitrogen atom has only one lone pair, limiting the extent of hydrogen bonding. Similarly, hydrogen fluoride is limited by having only one hydrogen atom available for bonding per molecule. Water, with its balanced number of hydrogen atoms and lone pairs, forms a more extensive and stronger hydrogen bond network, requiring more energy to disrupt and thus resulting in a higher boiling point. Comparing the boiling points of water, ammonia, and hydrogen fluoride directly reflects the effectiveness of their hydrogen bonding networks. Water’s higher boiling point, despite having a lower molar mass than HF, underscores the impact of its more extensive hydrogen bonding capability.
Hydrogen Bonding in Complex Molecules and Boiling Point Implications
Hydration of Ions
When ionic substances dissolve in water, water molecules surround the separated ions—a process known as hydration. Water molecules typically bond to positive ions via coordinate (dative covalent) bonds and to negative ions through hydrogen bonds.
Figure 5: Illustration of hydrogen bonds forming between chloride ions and surrounding water molecules during hydration.
As shown with a chloride ion (Cl-), water molecules orient themselves so that their (delta+) hydrogen atoms are attracted to the negative charge of the chloride ion and form hydrogen bonds with the lone pairs of electrons on the chloride ion. Even though lone pairs on larger halide ions like chloride are at higher energy levels (3-level in chloride), the strong negative charge of the ion enhances their ability to act as hydrogen bond acceptors. This hydration process, driven by hydrogen bonding, is crucial in dissolving ionic compounds in water. However, in the context of boiling points of pure substances, this effect is less directly relevant, as we are comparing boiling points of molecular substances, not ionic solutions.
Hydrogen Bonding in Alcohols vs. Ethers
Alcohols, characterized by the -OH group, and molecules with N-H groups, are capable of hydrogen bonding, significantly influencing their boiling points. Molecules capable of hydrogen bonding exhibit considerably higher boiling points compared to molecules of similar size and molar mass that lack -O-H or -N-H groups. This is because hydrogen bonding increases intermolecular “stickiness,” requiring more energy (heat) to separate the molecules and transition them into the gaseous phase.
Consider ethanol ((ce{CH3CH2-O-H})) and methoxymethane (dimethyl ether, (ce{CH3-O-CH3})), both having the same molecular formula ((ce{C2H6O})).
Ethanol possesses a hydrogen atom directly bonded to oxygen, allowing for intermolecular hydrogen bonding. Methoxymethane, while having an oxygen atom with lone pairs, lacks a hydrogen atom directly bonded to oxygen; its hydrogens are bonded to carbon, which is not electronegative enough to create a significant (delta+) charge for hydrogen bonding.
Compound | Boiling Point (°C) | Hydrogen Bonding |
---|---|---|
Ethanol ((ce{CH3CH2OH})) | 78.5 | Yes |
Methoxymethane ((ce{CH3OCH3})) | -24.8 | No |
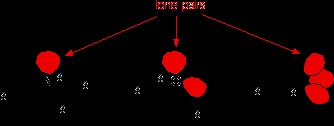
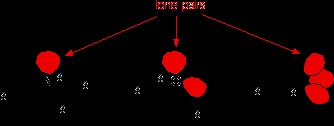
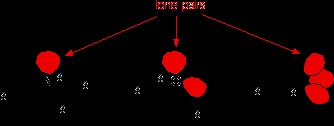
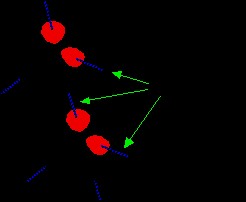
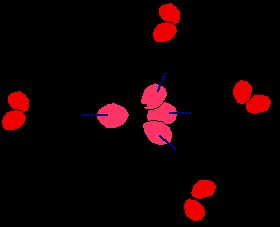
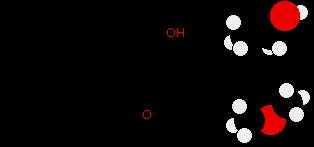
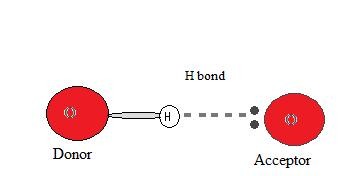
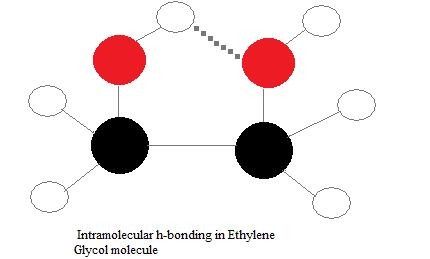
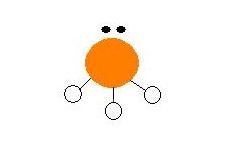
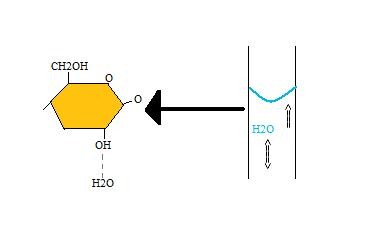
The stark difference in boiling points—over 100°C—clearly illustrates the dramatic impact of hydrogen bonding. Ethanol’s higher boiling point is directly attributable to the energy needed to overcome hydrogen bonds in addition to van der Waals forces. Methoxymethane, lacking hydrogen bonds, only relies on weaker van der Waals forces.
Comparing butan-1-ol, 2-methylpropan-1-ol, and diethyl ether further demonstrates this. Butan-1-ol, a straight-chain alcohol, exhibits a higher boiling point than 2-methylpropan-1-ol, its branched isomer, and diethyl ether, an isomer lacking hydrogen bonding, despite all having similar molar masses.
Compound | Boiling Point (°C) | Hydrogen Bonding | Molecular Structure Effect |
---|---|---|---|
Butan-1-ol | 118 | Yes | Straight chain |
2-methylpropan-1-ol | 108 | Yes | Branched chain |
Diethyl ether | 34.5 | No | Ether |
Both butan-1-ol and 2-methylpropan-1-ol have high boiling points due to hydrogen bonding, but butan-1-ol’s higher boiling point compared to 2-methylpropan-1-ol is due to stronger van der Waals forces in the straight-chain isomer. Branching in 2-methylpropan-1-ol reduces the efficiency of van der Waals interactions, slightly lowering its boiling point even with hydrogen bonding present. Diethyl ether, incapable of hydrogen bonding, has the lowest boiling point, showcasing the dominant role of hydrogen bonds in elevating boiling points when present.
Hydrogen Bonding in Nitrogen-Containing Organic Molecules
Similar to alcohols, organic molecules with N-H groups, such as amines, also exhibit hydrogen bonding, influencing their boiling points. This effect is crucial in larger biomolecules like proteins and DNA. In DNA, hydrogen bonds between nitrogenous bases on opposite strands of the double helix are critical for maintaining its structure and stability. These bonds form between hydrogen atoms attached to nitrogen on one strand and lone pairs on nitrogen or oxygen atoms on the opposing strand.
Donors and Acceptors in Hydrogen Bonding
For hydrogen bonding to occur, both a hydrogen donor and a hydrogen acceptor must be present.
The hydrogen donor is typically a highly electronegative atom (N, O, or F) covalently bonded to a hydrogen atom. This atom polarizes the H-X bond, making the hydrogen partially positive.
The hydrogen acceptor is an electronegative atom in a neighboring molecule (or within the same molecule for intramolecular bonds) that possesses a lone pair of electrons. This atom attracts the partially positive hydrogen atom, forming the hydrogen bond.
The formation of a hydrogen bond is driven by the electrostatic attraction between the partially positive hydrogen and the lone pair of the acceptor atom. This interaction is a type of dipole-dipole attraction but is significantly stronger due to the high electronegativity difference and the small size of the hydrogen atom, allowing for close approach and strong interaction.
Types of Hydrogen Bonds and Their Impact on Boiling Points
Hydrogen bonds can be classified based on their location and interaction type:
Intramolecular Hydrogen Bonds
Intramolecular hydrogen bonds occur within a single molecule, forming when a hydrogen donor and acceptor are present in the same molecule and are spatially close enough to interact.
In molecules like ethylene glycol ((ce{C2H4(OH)2})), hydrogen bonding can occur between its two hydroxyl (-OH) groups. Intramolecular hydrogen bonds can affect boiling points, often slightly lowering them compared to similar molecules capable only of intermolecular hydrogen bonding. This is because intramolecular hydrogen bonds reduce the molecule’s ability to interact with other molecules, thereby decreasing intermolecular forces and lowering the energy needed for boiling. However, the effect on boiling point is generally less pronounced than that of strong intermolecular hydrogen bonding.
Intermolecular Hydrogen Bonds
Intermolecular hydrogen bonds form between separate molecules and are the primary type responsible for the significant elevation of boiling points in substances like water, alcohols, and ammonia. These bonds can occur between like molecules (e.g., water with water) or unlike molecules (e.g., between water and alcohol in a mixture), provided that both donors and acceptors are present. The strength and extent of intermolecular hydrogen bonding directly correlate with boiling point elevation. Substances with extensive intermolecular hydrogen bonding networks require more energy to overcome these attractions, resulting in higher boiling points.
Properties and Effects of Hydrogen Bonds on Boiling Point and Viscosity
Boiling Point
Generally, boiling points increase with molar mass due to increased dispersion forces. However, hydrogen bonding introduces a significant deviation from this trend.
Compound | Molar Mass (g/mol) | Normal Boiling Point (K) | Hydrogen Bonding |
---|---|---|---|
(H_2O) | 18 | 373 | Yes |
(HF) | 20 | 292.5 | Yes |
(NH_3) | 17 | 239.8 | Yes |
(H_2S) | 34 | 212.9 | No |
(HCl) | 36.4 | 197.9 | No |
(PH_3) | 34 | 185.2 | No |
Water, hydrogen fluoride, and ammonia exhibit significantly higher boiling points compared to hydrogen sulfide, hydrogen chloride, and phosphine, despite having similar or even lower molar masses. This is a direct consequence of hydrogen bonding in (H_2O), (HF), and (NH_3), which are absent in (H_2S), (HCl), and (PH_3). Furthermore, water’s boiling point is higher than HF, even though HF has a slightly higher molar mass. This is attributed to water’s ability to form more hydrogen bonds per molecule than HF, creating a more interconnected network.
Comparing Boiling Points and Hydrogen Bond Strength: To compare the effect of hydrogen bonding on boiling points:
- Identify potential for hydrogen bonding: Look for molecules with H-N, H-O, or H-F bonds.
- Compare molar masses: If molar masses are similar, differences in boiling points are likely due to hydrogen bonding.
- Assess hydrogen bond network potential: Consider the number of hydrogen donors and acceptors per molecule. Molecules like water, with multiple donors and acceptors, form more extensive networks and have higher boiling points.
- Compare boiling point data: Analyze experimental boiling point values to quantify the impact of hydrogen bonding. Larger differences in boiling points between molecules with and without hydrogen bonding indicate stronger hydrogen bond effects.
Viscosity
Viscosity, the resistance to flow, is also affected by hydrogen bonding. Substances capable of forming hydrogen bonds tend to have higher viscosities because the intermolecular attractions hinder molecular movement. The more extensive the hydrogen bonding network, the higher the viscosity. For instance, substances like glycerol, with multiple -OH groups, exhibit high viscosity due to extensive hydrogen bonding.
Factors Limiting Hydrogen Bonding and Boiling Point
Electronegativity
A significant electronegativity difference between hydrogen and the atom it is bonded to is essential for hydrogen bonding. Molecules like phosphine ((PH_3)) do not exhibit hydrogen bonding because the electronegativity of phosphorus (2.1) is similar to that of hydrogen (2.1), resulting in a nonpolar P-H bond and no partial positive charge on hydrogen.
Without a significant dipole moment, the hydrogen atom in (PH_3) cannot form a hydrogen bond. Comparing (PH_3) to (NH_3) highlights this effect; ammonia’s higher boiling point is due to hydrogen bonding enabled by the high electronegativity of nitrogen, whereas phosphine’s lower boiling point reflects the absence of hydrogen bonding due to the low electronegativity of phosphorus.
Atom Size
The size of the donor and acceptor atoms can also influence hydrogen bond strength. Larger atoms may result in weaker hydrogen bonds due to reduced proximity between nuclei. For example, while chlorine is electronegative, hydrogen bonding involving HCl is weaker than with HF due to the larger size of chlorine compared to fluorine. This size difference affects the effectiveness of orbital overlap and charge density at the interaction site.
The Crucial Role of Hydrogen Bonding in Nature
Hydrogen bonding is fundamental to many biological processes and natural phenomena, most notably the unique properties of water. In plants, the cohesion-adhesion theory of water transport relies on hydrogen bonding for capillary action and water movement through xylem vessels.
Water molecules hydrogen bond to each other (cohesion) and to the cellulose walls of plant cells (adhesion), enabling water to ascend from roots to leaves against gravity.
In proteins, hydrogen bonds are crucial for secondary and tertiary structures. They stabilize alpha-helices and beta-sheets in secondary structure and contribute to the overall three-dimensional folding of proteins in tertiary structure. In DNA, hydrogen bonds between base pairs are essential for maintaining the double helix structure and genetic information integrity.
References
- Brown, et al. Chemistry:The Central Science. 11th ed. Upper Saddle River, New Jersey: Pearson/Prentice Hall, 2008.
- Chang, Raymond. General Chemistry:The Essential Concepts. 3rd ed. New York: Mcgraw Hill, 2003
- Petrucci, et al. General Chemistry: Principles & Modern Applications. 9th ed. Upper Saddle River, New Jersey: Pearson/Prentice Hall, 2007.